CENTURI Research groups
CENTURI Research groups
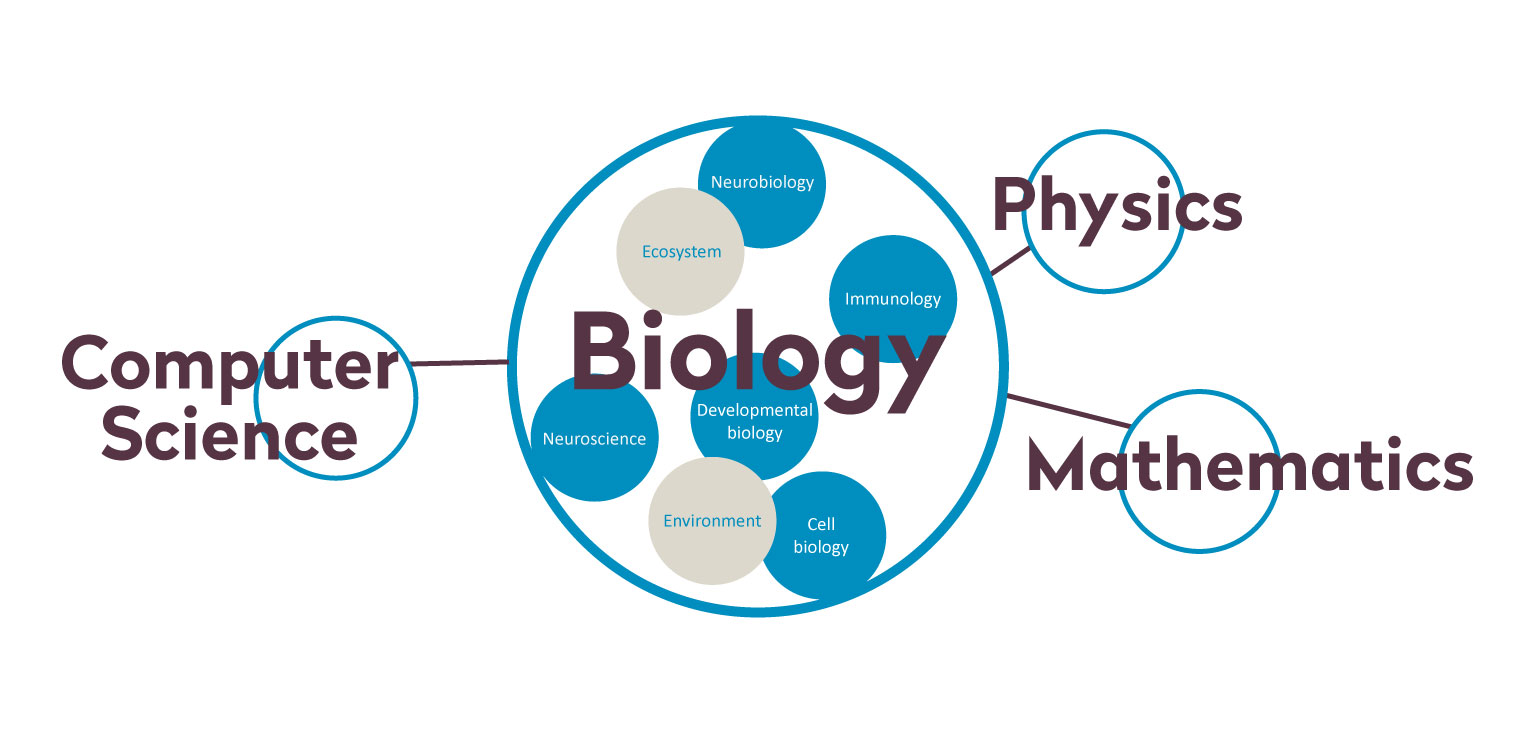
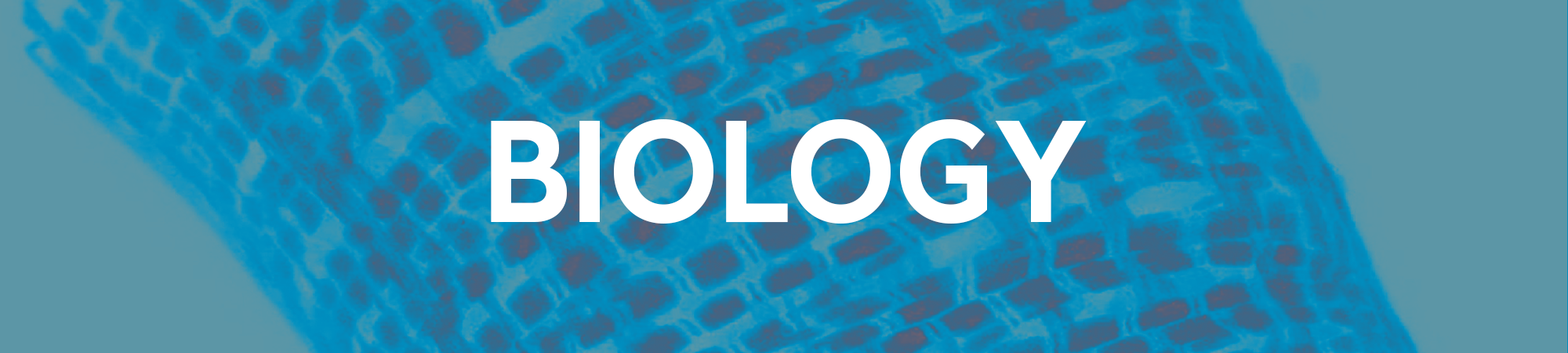
Architecture et Fonction des Macromolécules Biologiques (AFMB)
Campus / location: Luminy
Website: http://www.afmb.univ-mrs.fr/en/team/viral-macromolecular-complexes/
Team leader : Juan Reguera
Research domain: Microbiology, immunology, virology
Contact : Juan Reguera - Juan.Reguera@afmb.univ-mrs.fr
Our team focuses on understanding viral infection mechanisms often ruled by the interplay of viral proteins, cellular proteins and nucleic acids assembled into multifunctional complexes. The aim is to understand the mechanisms by which the multiple enzymatic activities are coordinated between them and regulated to efficiently carry out infection. To reach our goals we use a broad range of biochemical and biophysical technics combined with X-ray crystallography and electron microscopy.
The mainstream of our research is the functional and structural characterization of the alphavirus replication complex. Alphavirus, like chikungunya virus (CHIK) or eastern equine encephalitis virus (EEEV), cause zoonotic infectious diseases with high impact in global human health. The viral genome replication and gene expression is carried out by the multifunctional viral replication complex. Interestingly this complex can adopt different functions depending on its state of maturation. Our goal is to structurally and functionally characterize the different functional states of the replication complex in order to propose an integrative mechanistic model accurately describing at the molecular level the viral infection cycle and its regulation. The results will provide invaluable insights and tools for the development of new strategies for the treatment of these concerning infectious diseases.
Centre d'Immunologie de Marseille-Luminy (CIML)
Campus / location : Luminy
Website : http://www.ciml.univ-mrs.fr/science/lab-marc-bajenoff/home
Team leader : Marc Bajénoff
Research domain : Fundamental research in immunology
Contact : Marc Bajenoff - bajenoff@ciml.univ-mrs.fr
The immune system is composed of billions of motile immune cells that continuously patrol our organism and defend it against pathogens. The immune response takes place in dedicated organs such as lymph nodes (LN) or spleen. These organs are composed of 95% white blood cells and 5% of sessile stromal cells that form the skeleton of these organs. Without these architectural stromal cells, lymphoid organs would not exist and the entire immune responses would be compromised. Our laboratory is studying these stromal cells and seeks to understand their function, origin and dynamics.
To this aim, we are using several “multicolour fluorescent reporter” mouse models. In these mice, stromal cells stochastically acquire a color upon a given treatment (green, red, blue or a mixture of each color). This choice is random and genetically encoded, which means that every labelled cell transfers its color to its progeny. When a cell divides multiple times locally, it creates a monocolor cluster of cells around it (see figure below) and helps us understanding (i) where the cell has divided in the tissue, (ii) if the cell has divided few or many times (depending on the size of the cluster), (iii) if all the cells of a given lineage divide or not, (iv) if some cells give rise to other cell types (eg: if a cell type A creates a cluster that also contains cell type B, it means that cell type A could be the progenitor of B).
Campus / location : Luminy
Website : http://www.ciml.univ-mrs.fr/science/lab-marc-dalod/home
Team leader : Marc Dalod
Research domain : Systems biology, immunology
Contact : Marc Dalod - dalod@ciml.univ-mrs.fr
Our work is mainly focused on understanding the identity (cell type) and functions (cell states) of a family of immune cells called mononuclear phagocytes.
Mononuclear phagocytes are endowed with tissue maintenance and repair, microbicide, pro-inflammatory, antigen presentation or immunoregulatory functions. Therefore, these cells play critical roles in the initiation, functional polarization, maintenance and termination of immune responses. Mononuclear phagocytes encompass monocytes, macrophages and dendritic cells. Monocytes are mainly viewed as pro-inflammatory cells as well as precursors of macrophages. Macrophages are primarily endowed with tissue maintenance and repair or microbicide functions. Dendritic cells are the most potent cells of the body for antigen presentation and in particular for the activation of naïve T lymphocytes. However, there is further heterogeneity as well as functional plasticity with each of these subfamilies of cells. Different cell types can be identified within monocytes, macrophages or dendritic cells, which are characterized by the expression of a conserved core gene expression program and by a higher efficiency for specific functions. However, depending on the context, i.e. on its activation state, each type of mononuclear phagocyte can exert any of the functions mentioned above.
Currently, a big challenge in the study of the biology of mononuclear phagocytes is to rigorously identify cell types and cell states, in order to properly associate specific functions with well-defined combinations of cell types and cell states. This is a critical pre-requisite to be able to manipulate mononuclear phagocytes in the clinic to promote health over disease in many physiopathological contexts including infections, cancer and autoimmune or inflammatory diseases.
To address this issue, we are developing an integrated approach going through three major steps. The first step consists in using comparative genomics to identify cell types and cell states, by comparing the gene expression programs of cells isolated ex vivo from various tissues and several animal species under different activation conditions. We need to collaborate with bioinformaticians and mathematicians or physicists to analyze, interpret and model our data, in order to refine criteria to identify cell types versus cell states, and to predict potential functions and their molecular regulation for each combination of cell type and cell state. The second step consists in engineering and interrogating mutant mouse models to test the hypotheses generated in the first step, with the aim to characterize the orchestration of immune responses in time and space. This will involve perturbing the system through depletion of specific cell types, impeding the triggering of particular signaling pathways, or interfering with the induction of given cell states, and measuring how this affects immune responses. We will need collaboration with bioinformaticians and mathematicians or physicists for i) the identification and quantification of cell types and cell states on static tissue section or dynamically for intravital imaging analysis, and ii) integrating data from steps one and two, to model cellular interactions in time and space and their molecular regulation. The third step consists in testing whether the most interesting results obtained in mouse in the second step hold true for the functioning of the immune system of primates.
Campus / location: Luminy
Website: http://www.ciml.univ-mrs.fr/science/lab-mauro-gaya/b-cell-immunity-infection
Team leader: Mauro Gaya
Research domain: Immunology, B cells, Infection, Antibodies, Mucosas, Imaging
Contact: Mauro Gaya - gaya@ciml.univ-mrs.fr
B cells constitute essential elements of our immune system as they provide protection from pathogenic threats. The initial recognition of pathogens by B cells takes place in secondary lymphoid organs, such as the spleen and lymph nodes. Following pathogen encounter, B cells can enter into germinal center reactions, where they undergo sequential rounds of diversification and selection, resulting in the production of antibodies with high-affinity to the invading pathogen.
By using a wide range of techniques, from two-photon microscopy and multiparametric flow cytometry to mouse genetics and in vivo infection models, we study how the lymph node microenvironment controls the magnitude of antibody responses. Deciphering how and when those protective antibodies are generated and the immune network regulating their production, is central to develop alternative strategies for vaccination.
Campus / location : Luminy
Website : http://www.ciml.univ-mrs.fr/science/lab-jean-pierre-gorvel/home
Team leader : Jean-Pierre Gorvel
Research domain : Immunology, microbiology, vaccine
Contact : Jean-Pierre Gorvel - gorvel@ciml.univ-mrs.fr; Stéphane Méresse - meresse@ciml.univ-mrs.fr
The complex immune system of mammals is the result of co-evolutionary forces that include battles against pathogens, as sensing and defeating intruders is a prerequisite to host survival. The various microorganisms have evolved multiple mechanisms to evade both arms of the immunity: the innate and the adaptive immune systems.
The main purpose of our project is the depiction of the alteration of the dendritic cell membrane after its association with cyclodextrin molecules.
Projects:
- Five years ago, we developed a research program on the impact of cyclodextrines of bacterial origin on the immune system. The cyclic glucan of Brucella turned out to be a strong activator of dendritic cells (Martirosyan et al., PloS Pathogens, 2012). It is the basis for an interesting research programme since cyclic dextrans may have promise as a new generation of adjuvants. Previous observations in our lab revealed a key role for cyclic β–1,2–glucans (CβG) in procuring a full virulence to Brucella On the basis of its structural similarity to cyclodextrin molecules, which are efficient at separating cholesterol from biological membranes, CβG was also shown to interact with cholesterol on lipid-rafts (Arellano et al., Nat Immunol, 2005). Moreover, it is proven that the diminution or reorganisation of cholesterol produces a complete remodelation and change in plasma membrane dynamics, while signal transduction pathways are usually altered. We used fluorescence correlation spectroscopy (FCS), a technique especially suited for characterizing the dynamic behaviour of diffusing molecules across the cell membrane. Afterwards, the heterogeneity in diffusion can be deduced from diverse domain organizations, by applying a diffusion law to average temporal data. In the frame of CenTuri, we would like to create a model of CβG/plasma membrane interaction and have access to biophysical parameters of this interaction.
- Salmonella is an intracellular bacterium. It replicates inside host cells in a membranous compartment named the Salmonella-containing vacuole. This vacuole is characterized by the presence of membrane tubules that emerge from it and extend towards the cell periphery along microtubules. These tubules are formed by the action of Salmonella effector proteins. These proteins are injected by the bacterium into the infected cell cytosol. They behave as intracellular toxins by reprogramming the cell and favouring the replication of the bacterium. Considering the importance of these tubules in Salmonella virulence, it is important to understand how these tubules form, are stabilized and their function. The aim of this project is to develop a minimal in vitro system that reconstitutes the membrane tubulation that is observed in infected cells and controlled by Salmonella effectors. This system involves artificial membrane vesicles as biomimetics of the bacterial vacuole, recombinant Salmonella effectors and host components (microtubules and cytosol). Tubulation and membrane deformations are recorded by fluorescence microscopy (time-lapse, confocal) and atomic force microscopy.
Campus / location : Luminy
Website : http://www.ciml.univ-mrs.fr/science/lab-hai-tao-he-didier-marguet/home
Team leader : Hai-Tao He & Didier Marguet
Research domain : Cell membranes, signal transduction, lymphocytes, molecular diffusion, biophotonics, fluorescence correlation spectroscopy, super-resolution microscopy
Contact : Hai Tao He - he@ciml.univ-mrs.fr; Didier Marguet - marguet@ciml.univ-mrs.fr
The membrane (i.e. the plasma membrane, PM) delineating the interior of individual living cells serves as physical barrier to the environment, creating the conditions to locally concentrate molecular constituents. It is also the site in which mechanistic processes occur to keep a cell interconnected with its environment. This membrane is composed of constituents that interact with each other to form a dynamic two-dimensional fluid displaying significant lateral heterogeneity on different spatiotemporal scales. This is thought to have important functional contributions, in particular to the surface receptors that are expressed on the plasma membrane and mediate the transduction of information.
► An understanding of the biophysical principles that govern the lateral organization and dynamics of the plasma membrane is essential in understanding how the information is initiated at the plasma membrane to end up the nucleus by modifying specific gene expression.
Specifically, we are interested to characterize the contribution of plasma membrane lateral dynamics and organization in living lymphocytes and during signal transduction processes.
Based on biophotonics, we aim at making quantitative description of the dynamics of the different molecular players at the plasma membrane and how they are involving in and regulated during the course of T cell activation at both the molecular and cellular scales. The observables will require modeling in collaborations with mathematicians and physicists in order to conceptualize an appropriate understanding of the processes.
Our studies therefore fit into two main axes:
- At the molecular level, our experiments examine at high spatial and temporal resolution the molecular dynamics and interactions at the plasma membrane of living T cells by using the state-of-the-art biophotonics methodologies.
- At the cellular level, we investigate the mechanisms allowing T cell to integrate sequential and transient signals while interacting with the antigen presenting cells, critical for the decision making: the commitment to T cell activation program. Here, the experimental approaches will combine biophotonics, optogenetics and microfluidics.
Campus / location : Luminy
Website : http://www.ciml.univ-mrs.fr/fr/science/lab-magali-irla/immune-tolerance-and-t-cell-differentiation
Team leader : Magali Irla
Research domain : Fundamental research in immunology
Contact : Magali Irla - irla@ciml.univ-mrs.fr
T cells are essential for effective immune defense against tumor cells and pathogens, including viruses, bacteria and fungi. These cells undergo a strict developmental program in the thymus that leads to the generation of a self-tolerant T-cell repertoire capable of responding to foreign antigens. Physiologically, the thymus undergoes profound changes due to age-related involution, which results in decreased T-cell production, thus increasing the risk of infection, cancer and autoimmunity. Importantly, T-cell development and selection are dictated by thymic epithelial cells (TECs) that constitute a major component of stromal niches. Reciprocally, developing T cells control TEC differentiation and organization, a process referred to as “thymic crosstalk”. Single-cell transcriptomic analyses have revealed that TECs are highly heterogeneous with a dozen of subsets endowed with distinct molecular and functional properties.
By using several cutting-edge technologies such as single-cell RNA-seq, spatial transcriptomics, multi-parametric flow cytometry and imaging approaches applied to unique transgenic mouse models, our team aims to unravel the cellular crosstalks responsible for T-cell tolerance induction. Understanding the mechanisms underlying the thymic function is expected to pave the way for therapeutic strategies to treat autoimmune diseases and restore T-cell production during aging.
Campus / location : Luminy
Website : http://www.ciml.univ-mrs.fr/science/lab-pierre-milpied/integrative-b-cell-immunology
Team leader : Pierre Milpied
Research domain : Developmental immunology
Contact : Pierre Milpied - milpied@ciml.univ-mrs.fr
Antibodies are lifesaving biomolecules – major effectors of vaccines efficacy and powerful therapeutics for cancer treatment. In natural immunity, highly potent antibodies are produced by the descendants of a single antigen-specific B cell after complex processes of cellular and molecular maturation. In the aim of designing new therapies for challenging infections and cancers, our laboratory gathers expertise in cellular immunology, single-cell genomics, and bioinformatics, to decode B cell responses in health and disease.
Campus / location : Luminy
Website : http://www.ciml.univ-mrs.fr/science/lab-bertrand-nadel/home
Team leader : Bertrand Nadel
Research domain : Cancer Science, immunology, genetics
Contact : Bertrand Nadel - nadel@ciml.univ-mrs.fr; Pierre Milpied milpied@ciml.univ-mrs.fr; Dominique Payet-Bornet - payet@ciml.univ-mrs.fr; Delphine Potier - potier@ciml.univ-mrs.fr
Group 1 (P. Milpied): Integrative single-cell analysis of normal and neoplastic B cells
We study normal immune cells (B lymphocytes) that are involved in protection against pathogens after infection or vaccination. We also study the cancer cells (lymphoma) that derive from B lymphocytes. We develop single-cell analysis tools to model and quantify those cellular systems in in vivo mouse models and human patients’ samples.
We aim to gather gene expression measurements at single-cell resolution and to integrate those data to other biological data (protein expression, genetic mutations) and metadata (time after stimulus, location of cell). We use those data to model and quantify the biological behavior of our cell types of interest.
We are particularly interested in algorithmic methods for dimensionality reduction, differentiation trajectory inference, sparse data analysis, data integration, quantitative agent-based modeling.
Group 2 (D. Payet-Bornet; D. potier): T-cell acute lymphoblastic leukemia (T-ALL)
We decipher mechanisms involved in T-ALL oncogenesis. To do so, we develop and analyze T-ALL mouse models and generate Patient derived xenografts.
We aim to determine how cell-intrinsic genetic defaults in T-cells (genomic, epigenetic) lead to malignant transformation. More recently we investigated the impact of cell-extrinsic cues, notably the role that the T-Cell Receptor (TCR) might play in leukemogenesis.
We are interested in the analysis of transcriptional regulation, developmental regulatory networks and epigenetic disruptions. We are also modeling TCR signaling network.
Campus / location : Luminy
Website : http://www.ciml.univ-mrs.fr/fr/science/lab-philippe-naquet/introduction
Team leader : Philippe Naquet
Research domain : Immunity and inflammation
Contact : Philippe Naquet - naquet@ciml.univ-mrs.fr
We are working in the field of tissue immunity and its application to understanding disease evolution such as inflammatory diseases and cancer. Our basic question is to understand how a tissue copes with a chronic stress imposed by a pathology and how immune responses adapt their behavior to the chronically damaged tissue, raising the issue of communication modes between two major cell compartments: tissue and infiltrating immune cells.
Stress imposes severe constraints to the tissue forcing it to handle tissue damage, to induce repair processes while preserving its functional activity. Metabolic adaptation is critically involved in this regulation. We have identified a novel metabolic pathway involving Vnn genes and linked to vitamin B5 which controls its bioavailability in tissue. Vitamin B5 is the precursor of coenzyme A in cells, and therefore at the origin of major energetic resources.
We showed in various pathologies that modulating the Vnn pathway changes the outcome of the pathology. Applied to a cancer models (soft tissue sarcoma), we showed that the expression of the Vnn1 isoform was associated with a good prognostic of tumor evolution due to its restricted growth potential, enhanced cell differentiation and improved anti tumoral immune response. When exploring the link between these phenotypes, we found that some are tumor-cell autonomous whereas other involve communication between tumor and immune cells, in part through the production of energetic metabolites able to act as second messengers (metabokines) for immunocytes. Therefore, by adapting their metabolic pathways, cells can either compete or collaborate for energetic resources and exchange numerous soluble signals including cytokines and metabokines which in the end condition disease evolution.
Modelling this complex signaling network by quantifying a fraction of these molecular signatures and their interplay should allow a better prediction of tumor evolution.
Campus / location : Luminy
Website : http://www.ciml.univ-mrs.fr/science/lab-philippe-pierre/home
Team leader : Philippe Pierre
Research domain : Immunology
Contact : Philippe Pierre - pierre@ciml.univ-mrs.fr
In the blood, mucous membranes and lymphoid organs, dendritic cells provide a dual role as sentinels, but also as conductors of the immune orchestra. Hiding in the entryways used by pathogens, dendritic cells locate infectious agents, ingest them and release biochemical signals to alert the first line of defensive cells in the body and draw them to the site of the infection. Once the intruder has been digested, dendritic cells also expose on their their surface fragments of the pathogen: the antigens. They then migrate via the lymphatics to secondary lymphoid organs (spleen, lymph nodes, mucosa-associated lymphoid tissue of the digestive tract and lungs) where they present these antigens to T and B lymphocytes. Once "armed" against the pathogen as a consequence of this process, these highly precise defenders in turn migrate to the site of infection to ensure its eradication.
In this sequence of events, the team of Philippe Pierre is particularly interested in the key stage of maturation of dendritic cells. This is the moment when the cells detect the microbes, change their biological functions and begin their migration to secondary lymphoid organs.
Campus / location : Luminy
Website : Innate immunity in C. elegans | CIML (univ-mrs.fr)
Team leader : Nathalie Pujol
Research domain : Fundamental research in skin immunology & developmental biology
Contact : Nathalie Pujol - pujol@ciml.univ-mrs.fr
Caenorhabditis elegans is a roundworm widely used by geneticists to study the development and function of living organisms. In 1998, it was the first multicellular animal whose genome sequence was published in full. The majority of human genes are known to have functional equivalents in this worm. Importantly, it is technically very easy to turn off or turn on a gene of C. elegans and to analyse the biological consequences of this manipulation. Because of this, many large-scale studies have been undertaken using C. elegans, and there is a wealth of publically available qualitative and quantitative functional data.
We use this "simplified model" to understand better the mechanisms of defence against infections by fungi and bacteria. We apply a broad range of techniques, from genetics, to biochemistry, cell biology, bioinformatics, and functional genomics, and have built up a detailed picture of innate immunity in the nematode. Very recently, we have expanded our research to include a molecular and cellular dissection of fungal virulence mechanisms. We are addressing the strategies deployed by the nematophagous fungus Drechmeria coniospora to infect C. elegans, and investigating co-evolution of pathogen virulence and host defences.
For the last decade, we have been attempting to incorporate large-scale and quantitative approaches to our work. As a consequence, we have long standing collaborations with mathematicians and bioinformaticians, including colleagues at the IML and TAGC, and participate in the Labex INFORM.
Campus / location : Luminy
Website : http://www.ciml.univ-mrs.fr/science/lab-romain-roncagalli/integrative-biology-t-cells
Team leader : Romain Roncagalli
Research domain : T cell biology, TCR signaling, Proteomics, Systems Biology
Contact : Romain Roncagalli - roncagalli@univ-mrs.fr
For years, the analysis of T cell signaling pathways has been limited by the experimental tools available, and often restricted to a limited number of proteins selected based on prior knowledge. Recent technological advances now allow to characterize protein networks and determine the abundance and post-translational regulation of almost each protein expressed in a cell. Our team develops original Mass Spectrometry (MS)-based approaches to generate comprehensive and dynamic models of the molecular processes that are associated with T-cell’s states.
Using genetically modified mouse models, we look at how proteins interact and change when T cells encounter different signals. In particular, we seek to characterize the stoichiometry and dynamics of protein-protein interactions (PPIs) encoding the engagement of the TCR and others receptors expressed on the cell surface of lymphocytes.
Using time-resolved high-resolution phosphoproteomics, we are also able to identify, quantify, and characterize the phosphorylation dynamics of thousands of phosphorylation sites in primary T cells after TCR stimulation. Our work reveals a coherent orchestration of biological processes and functional modules associated with cytoskeletal remodeling, transcription, translation, and metabolic processes in the early stages of TCR engagement. Using similar approaches, we also explore the molecular mechanisms that enables T cells to discriminate antigens of varying affinities.
In the course of our research, we have come to realize that the mechanisms regulating T cell signaling, and therefore their fate, are far more complex than originally thought, and that many molecular components essential to T cell regulation have not yet been characterized. Our aim is to assess this complexity at multiple scales, from the molecule, through the cell, to the living organism. Indeed, our research strategy consists in going back and forth between high-throughput methods and targeted molecular analysis to associate molecular regulations with cellular phenotypes that emerge under different physiological conditions.
Campus / location: Luminy
Website: http://www.ciml.univ-mrs.fr/science/lab-rejane-rua/immunosurveillance-central-nervous-system
Team leader: Rejane Rua
Research domain: Immunology, macrophages, Infection, Central Nervous System, Imaging
Contact: Rejane Rua - rua@ciml.univ-mrs.fr
The Central Nervous System (CNS) is protected by the meninges, a three-layer covering that provides a structure onto which a myriad of resident innate immune sentinel cells block threatening pathogens or activate the adaptive immune system in response to inflammatory challenges. The recent discovery of this complex and dynamic meningeal innate immunity means that the development of new targeted therapeutic agents for the treatment of neurological disorders is within the realm of possibility.
Our team is using cutting-edge technologies such as intravital imaging, histo-cytometry, functional flow cytometry and single-cell transcriptomic approaches that together allow for a precise and dynamic investigation of the meninges in wildtype and transgenic mice models. We aim to unravel the remarkable complexity and plasticity of the immune sentinels’ functions in health and disease.
Campus / location : Luminy
Website : http://www.ciml.univ-mrs.fr/science/lab-serge-van-de-pavert/home
Team leader : Serge van de Pavert
Research domain : Developmental immunology
Contact : Serge van de Pavert - vandepavert@ciml.univ-mrs.fr
Lymph nodes are important for the immune system to fight intruders and its formation already starts within the embryo with the interaction of 2 cell types at pre-destined locations. We are interested in the differentiation process of 1 of these involved cells, and analyze the expression of genes within these cells during subsequent steps of the differentiation process to obtain information on the requirements for differentiation. This requires the aid of bio-informaticians/ mathematicians to be able to compare the right sets of genes and obtain new knowledge on the pathways involved. Also, since these structures are always formed at exactly the same position within the embryo, cues from the surrounding must aid the attraction and differentiation of the cells involved. Based on previous studies, we postulated that neurons might be involved. We are testing this hypothesis by studying embryos in which the location of these neurons has been altered slightly. If the hypothesis is correct, changing location of the neuron should also alter the location of the lymph node. We have obtained the light sheet microscope, which allows 3D reconstructions of large fluorescently stained tissues and are able to acquire involved structures (see side view of a 1 cm large E13.5 mouse embryo in figure on the right; neurons= green, lymphatic structure= purple, cells in lymph node= yellow). However, we are looking for (mathematical) methods to analyze and quantify the changes in shape and location of the lymph node and lymphatics in a whole mount embryo, normalizing the location for small size differences of each embryo.
Modelling this complex signaling network by quantifying a fraction of these molecular signatures and their interplay should allow a better prediction of tumor evolution.
Our group is specialized in embryonic development of the immune system and ontogeny of a involved cell type. Our niche is in between immunology, neurobiology and developmental biology. We can assist with the analysis of cells within whole tissues and/or organs up to 1 cm3, e.g. embryos, tumors, brains, etc. We hope to discuss with- and get input from- participants of the CenTuri network on the profiling of these cells and to aid us in the calculations of the structures within the embryo. We ultimately aim to understand and form a model on how several cells can interact at a given space and time to form an organ.
Centre d'Immunophénomique (Ciphe)
Campus / location : Luminy
Website : http://ciphe.marseille.inserm.fr/en/
Team leader : Hervé Luche
Research domain : Cell Biology
Contact : Hervé Luche - herve.luche@inserm.fr ; Bernard Malissen - bernardm@ciml.univ-mrs.fr ;
Over the past decade, a major change in biology has occurred with the development of large-scale projects (large sequencing projects, intelligent databases for DNA genotyping, proteomics and metabolomics). Cell biology has followed the same trend, particularly with the latest developments in multiparametric flow cytometry (MFC) and mass cytometry (CMM). The performance of flow cytometers has been improved by using high-power LEDs for optimal excitation of fluorochromes over the full range of the light spectrum. The Helios (Fluidigm Inc) mass cytometer, a hybrid instrument between a flow cytometer and a mass spectrometer, has acquired up to 40 parameters at the single cell level. On the reagent side, the development of a series of new-order bright fluorochromes (fluorescent polymers of Syrigen type BV and BUV) in CMF as well as a chemistry allowing the coupling on antibodies of many rare earth elements in CMM, has opened the way to analyses with very high information content at the cellular level describe the cellular heterogeneity of a sample.
This massive increase in the number of markers studied simultaneously at the single cell level leads us to take a methodological step forward in data analysis. In order to reveal the most relevant cellular immuno-phenotype that can be correlated to the biological effect studied, the biologist is unable to use manual methods of supervised fenestration of events. He must move to unsupervised approaches to analysis in order to reduce the complexity of his dataset to the variations most relevant to his study. To this end, two main types of methods can be used, or even combined in an analysis pipeline. The events of one or more samples can be classified according to their phenotypic proximity in the multidimensional space (example: SPADE or Citrus algorithm). Variations in marker expression within the same cluster can then be studied between individuals. The other type of approach consists in using PCA or tSNE dimension reduction methods to redistribute events in two dimensions by taking into account their variability as markers used for clustering. Other methods based on machine learning approaches. It would be relevant to evaluate, in the context of the Centuri Institute, the implementation of other techniques in TDA (topological data analysis) to more effectively understand the structure of multi-parametric data as is being done in INRIA's Datashape initiative.
Most of the tools available for advanced data analysis of multiparameter cytometry datasets are developed in R language as software packages. In most cases, these tools must be combined in analytical pipelines to ensure data control, analysis and visualization of results. In order to improve the quality and speed of the immunophenotyping analyses proposed in our service activities, the CIPHE immunophenotyping module recruited a bioinformatician (Q. Barbier) to apply new analytical methodologies. However, due to the absence of biological interfaces, the advanced analyses established cannot be transferred to a large user base. This question is very critical, as the interpretation of this analysis is rather slow: biologists are not able to use these tools themselves, verify their results and study the biological significance of these new observations (Chattopadhyay PK, Am. Transplantation 2015). In order to unleash the power of unsupervised automated data analysis, new interfaces should be developed to provide R-scripts to the broader community of cytometrists in a "biologist-friendly" manner. The use of R-shiny, a web application interface for R scripts, analysis pipelines built in R language can be transformed into interactive web applications. This type of effort would benefit greatly from the input of mathematicians in the design of pipelines for methods applied to multiparametric data analysis.
In view of the growing needs in computational cytometry analysis, it also seems more than necessary to integrate an EU on methods of data analysis in automated multiparametric cytometry into a master's degree course in bioinformatics in Marseille.
Centre de Recherche de Cancérologie de Marseille (CRCM)
Campus / location : Marseille Cancer Research Center
Website : https://www.crcm-marseille.fr
Team leader : Patrick Chames
Research domain : Nanobodies, Immunotherapy, Multispecificity, Targeting
Contact : Patrick Chames - patrick.chames@inserm.fr
The ATI team explores the potential of nanobodies for the generation of innovative multispecific immunomodulators and the targeting of payloads for cancer immunotherapies.
The recent successes of therapeutic antibodies called immune checkpoint inhibitors (ICIs) blocking the inhibitory receptors found on tumor-infiltrated immune cells have revolutionized the therapy of several cancers, to the point that immunotherapy is now considered the fourth pillar of cancer therapy together with surgery, radio and chemotherapy. Still, only a minority of treated patients benefit from those treatments, and there is an urgent need to expand the arsenal of ICIs. Beside the classically targeted T cells, natural killer (NK) cells are reportedly important actors of the anti-tumor immune response, through the killing of tumor cells, but also and most importantly through the secretion of several cytokines and chemokines that can recruit and activate cells of the innate and adaptive immune systems.
The main interest of our lab is to use antibody engineering solutions to generate innovative molecules able to efficiently modulate the anti-tumor immune response through the recruitment and activation of immune cells using multispecific cell engagers or multispecific immune checkpoint inhibitors, or through the vectorization of radionuclides and nanoparticles.
Our team has developed a solid expertise in the generation and use of nanobodies, corresponding to the variable domain of antibodies naturally devoid of light chain found in camelids, for cancer therapy and diagnostic using in vitro selection methods. We are exploring the possibilities offered by this technology for the development of innovative cancer imaging and therapy approaches.
Campus / location : Institut Paoli Calmettes
Website : https://www.crcm-marseille.fr
Team leader : Estelle Duprez
Research domain : hematology, hematopoietic stem cell, aging, transcriptional network, epigenetic factors
Contact : estelle.duprez@inserm.fr
The main scientific objective of the team is to decipher the transcriptional networks involved in the regulation of proliferation versus differentiation of normal or pathological hematopoietic cells. We focus on the transcriptional and epigenetic mechanisms involved in age-related alterations of hematopoietic stem cells (HSCs) and their consequences in immune-senescence and myeloid diseases that occur in the elderly. One of our objectives is to identify the molecular and cellular parameters of HSC aging that influence the progression of pathologies of the hematopoietic system.
We use cellular and mouse models of HSC aging and generate transcriptomic and epigenomic data in bulk and single cell level. We are analyzing and integrating our data by taking into account the different levels of information we generate (chromatin, RNA, protein). We are also developing approaches to capture the spatial information of the bone marrow and integrate this information to study the communication of HSCs within their microenvironment and address the issue of age-related changes in the dialogue between two cellular entities.
Campus / location : Institut Paoli Calmettes
Website : https://www.crcm-marseille.fr
Team leader : Flavio Maina
Research domain : Signalling, cell-cell crosstalk, mouse models, tumoroids, -omics,
Contact : Flavio Maina - flavio.maina@univ-amu.fr
We explore signalling circuits operating during tumour initiation and progression and representing vulnerabilities of cancer cells, which may correspond to new putative therapeutic opportunities.
Using clinically relevant tumoroid and genetic models of liver or breast cancer, we conduct an interdisciplinary research combining -omics approaches with computational biology and mechanistic/functional studies, in order to design and evaluate combinatorial treatments targeting cancer and immune cells.
As cancer models: 1) we have generated clinically relevant genetic models that recapitulates several features of human liver or breast cancer patients (Alb-R26Met and MMTV-R26Met mice, respectively); 2) we employ hydrodynamic tail vein injection to generate a panel of complementary liver cancer models; 3) we use orthotopic injections of cancer cells and/or tumoroids in immune-competent syngeneic mice; 4) we established tumoroids (and control organoids) from liver and breast cancers. Furthermore, the team has built a network with clinicians and oncologists at the Institut Paoli-Calmettes and at St. Joseph Hospital (Marseille) to establish tumoroids from patient biopsies and/or resected tumours.
Campus / location : Institut Paoli Calmettes
Website : https://www.crcm-marseille.fr
Team leader : Mauro Modesti
Research domain : Mechanisms of DNA double-strand break repair
Contact : Mauro Modesti - mauro.modesti@inserm.fr
Our team concentrates on understanding DNA double-strand break repair mechanisms in human cells and other model systems by Homologous Recombination and Non-Homologues End Joining. To investigate these mechanisms we reconstitute these DNA repair machineries in vitro to study how they function at the molecular level using bulk and single-molecule assays. We also study pathological and physiological DNA double-strand break repair mechanisms in cells and are now aiming at visualizing the components of DNA repair machineries inside living cells using advanced microscopy techniques.
The results obtained from our research are of broad interest to those who study DNA repair and genome integrity maintenance, immune system development, cancer biology, neurobiology, and for development of genome editing technologies.
Institut de Biologie du Développement de Marseille (IBDM)
Campus / location : Luminy
Team leader : Vincent Bertrand
Research domain : Development, neurobiology, polarity, transcription
Contact : Vincent Bertrand - vincent.bertrand@univ-amu.fr
Our team is analyzing how the nervous system is built during animal development, and subsequently maintained during adulthood. To address this question we are using quantitative live imaging approaches and the invertebrate C. elegans as a model organism. More precisely, we are addressing two main questions:
1) Polarization of a field of neuronal precursor cells
Neurons are produced by the asymmetric divisions of neuronal precursor cells. We are trying to understand how the divisions of the neuronal precursors are regulated and coordinated in space. We have identified secreted ligands, receptors and intracellular transducers involved in this process. In collaboration with the team of Pierre-François Lenne (IBDM) we are currently using quantitative live imaging methods to analyze the dynamics of these molecular players during the polarization process in vivo. Interactions with modelers will be important in the future to integrate these quantitative data into a general model of tissue polarization.
2) Specification and maintenance of neuronal identity
A high diversity of neurons is generated during the development of an animal. These neurons subsequently maintain their identity throughout the life of the animal. Because defects would lead to the generation of an abnormal complement of neurons during development or to the progressive loss of some neuronal populations during aging, this process has to be strictly controlled. The identity of neurons is regulated by networks of transcription factors, but how these networks can ensure a reliable specification and maintenance of neuronal fate despite the noise present in gene expression and the variability in environmental conditions is unclear. To address this question we are using CRISPR based genome engineering approaches and live imaging to analyze the dynamics of a network of transcription factors involved in the regulation of neuronal cell fate. Collaborations with modelers will be important to build a model of this network and to better understand its response to perturbations such as physiological stresses.
Campus / location : Luminy
Website : http://www.ibdm.univ-mrs.fr/equipe/molecular-control-of-neurogenesis/
Team leader : Harold Cremer
Research domain : Development, neurobiology
Contact : Harold Cremer - harold.cremer@univ-amu.fr
Understanding neural stem cell diversity and differentiation at the molecular level: Genes and microRNAs
A key question in developmental neurobiology concerns the molecular mechanisms that control the specification of stem cells to produce neurons with defined neurotransmitter phenotype, positions and connectivity. Combinatorial expression of transcription factors, highly controlled in space and time, has been shown to underlie the determination events that lead to neuronal diversity. Historically these endogenous factors have been considered to act in an « all-or-nothing » fashion. However, it is now clear that many of these factors act highly dose-dependent.
Using adult neurogenesis in mice as the experimental model we found that small differences in transcription factor concentration in neighboring neural stem cells, fine-tuned by the superposed action of specific regulatory non-coding RNAs (microRNAs), lead to distinct neuronal fates. Moreover, neuronal fate decisions are not restricted to the stem cell compartment, but can occur at later stages of neuronal differentiation.
To systematically investigate the expression, interaction and function of mRNAs and microRNAs during adult neurogenesis in space (in different stem cell populations) and in time (from stem cells to neurons).
Based on our ability to genetically label and isolate defined neural stem cell populations and their entire lineages in vivo, we generated unique high-resolution gene- and microRNA expression data. While initial studies have been performed at the cell-population level - labeling defined stem cell pools and investigating their transcriptome/microRNome as a whole - it is now clear that we have to perform such analyses at higher resolution, using new technologies like single cell RNAseq.
Campus / location : Luminy
Website : https://www.ibdm.univ-amu.fr/team/epithelial-monolayer-morphogenesis/
Team leader : Delphine Delacour
Research domain : Epithelium – Intestine – Organoids – Tissue morphogenesis and integrity - Cytoskeleton
Contact : Delphine Delacour - delphine.DELACOUR@univ-amu.fr
Epithelia act as a physical barrier against external aggressions but also ensure organ functionality. If not correctly assembled or dysfunctional, this leads to pathological situations that include a variety of diseases spanning from rare developmental syndromes or cancer. How epithelia coordinate and harmonize the responses of each cell with not only their nearest neighbors, but entire tissue, to guarantee proper spatial arrangement, integrity and functionality is still not well understood. So far, the question of epithelial coherence has been mainly tackled in invertebrate models during development or in transformed cell lines in culture.
The intestinal epithelium is a tremendous model for this field of research, as it is one of the most fast-proliferative and regenerative in mammalian organisms. In addition, the intestinal tissue is subject to adverse situations, where the balance between cell proliferation, differentiation and death has to be strictly maintained. However, the study of the cellular and developmental mechanisms that govern the development and architecture of the intestinal tissue is still in its infancy.
The general objective of our project focuses on understanding the determination and maintenance of intestinal functional domains, and to evaluate their spatiotemporal coordination. More specifically, our aims are: 1) to define mechanisms controlling the integrity of the proliferative domain, and assess their impact on the crypt development; 2) to characterize the epithelial connectivity and collective behavior of the differentiated domain in homeostatic conditions or under challenging contexts.
One of the strong aspects of our research is to confront in vivo and in vitro murine and human disease models, and to combine different approaches from advanced cell biology, tissue engineering, histology, molecular biology, biophysics and computational modelling. This strategy allows us to determine the adaptive processes that epithelial cells employ to polarize and organize in a given environment, and enhance their ability to modulate their fate.
To know more:
1- Cryptogenesis
Research on intestinal crypts has mostly focused on stem cell asymmetric divisions in terms of fate or rate of proliferation. However, crypt morphogenesis by itself remains an understudied field of research. In recent studies, we have implemented intestinal organoid cultures, their high-resolution imaging and genetic manipulations to decipher the cryptogenesis process. We currently address mechanisms regulating the crypt organization, and their contribution to the development of rare intestinal diseases and cancers.
2- Epithelial organization and connectivity
How is ensured multicellular coordination in mammalian adult epithelia? We assess cell adhesion patterning and supracellular network emergence at the tissue level in vitro in organoid cultures and in vivo in mammalian tissues, and determine their spatiotemporal orchestration for epithelial connectivity.
Campus / location : Luminy
Website : http://www.ibdm.univ-mrs.fr/equipe/stem-cells-and-brain-repair/
Team leader : Pascale Durbec
Research domain : Neurobiology, brain plasticity
Contact : Pascal Durbec - pascale.durbec@univ-amu.fr
Despite the existence of reservoir of stem cells and progenitors, the central nervous system shows really limited regenerative properties after lesion or in neurodegenerative conditions. An important issue in neuroscience is to characterize these stem/progenitor cell populations in physiological and pathological contexts and to design future cell based therapeutic strategies to promote brain regeneration. A spontaneous regenerative process involving the production of new mature oligodendrocytes (OLG) the myelin forming cells of the brain, has been observed after demyelination in Multiple Sclerosis patients. This process is rarely effective and the loss of myelin (demyelination) is accompanied by a disruption in the ability of the nerves to conduct electrical impulses to and from the brain. The objective of the team is to describe this regenerative process in rodent.
Stem and precursor cells of the adult Sub-ventricular zone (SVZ) are implicated in this spontaneous regenerative process. In physiological conditions, SVZ stem cells generate mainly neuronal progenitors, which migrate to the olfactory bulb, where they differentiate into interneurons. Several studies have shown that in demyelinating conditions, some SVZ-derived neuronal progenitors are mobilized and migrate toward lesions. This surprising observation changes our vision of cell commitment and raised important questions concerning post-lesional cell plasticity in the adult brain: how a cell committed to a specific lineage can change fate? Can neuronal progenitors generate OLG by transdifferentiation or do they need to first de-differentiate to change fate? What are the molecular mechanisms regulating this fate switch in the adult brain?
We have strong preliminary showing that a few days after demyelination, neuronal progenitors generate OLG through direct transdifferentation. Indeed, we observed around the lesion and in the SVZ a population of cells, that we called “the mixed entity”, expressing concomitantly both neuronal and OLG markers. Our objectives are to extend our knowledge on in vivo spontaneous cell transdifferentiation events by comparing the transcriptional signatures of the mixed entity to that of neuronal progenitors and of OLG in physiological conditions and after lesion. This analysis based on single cell transcriptomic analysis should provide insight into the molecular cascades controlling in vivo direct cell reprogramming.
In a short term, we will benefit from interaction with mathematicians to theoretically describe and model this cell reprogramming even. Based on single cell transcriptomic data, we could perform modeling and machine learning techniques for the analysis of gene regulatory networks to map the landscape between the dynamic cellular states identified during in vivo cell reprogramming and to model the genomic modifications due to lesion induction.
Campus / location : Luminy
Website : http://www.ibdm.univ-mrs.fr/equipe/molecular-mechanisms-underlying-mesenchymal-cell-differentiation/
Team leader : Laurent Fasano
Research domain : Developmental biology
Contact : Laurent Fasano - laurent.fasano@univ-amu.fr
As a developmental biologist, I aim to understand how cells initially identical become different by expressing different set of genes.
Decoding the temporal control of gene expression patterns is key to the understanding of the complex mechanisms that govern developmental decisions during organ/tissue development. My team has identified the teashirt (Tshz) genes family that encode evolutionarily conserved zinc finger transcription factor (TSHZ). Our research centres on understanding how TSHZ3-related transcriptional networks help cells taking on gradually a final identity within the context of normal and pathological development.
Recently, we identified TSHZ3 as the critical gene for a syndrome whose characteristic clinical manifestations are autism spectrum disorders and congenital anomalies of the kidneys.
To identify the key mechanisms that contribute to the initiation, development and maintenance of autistic-type phenotypes along the temporal axis we perform a multilevel study, from behaviour to molecule, using mouse models. At the molecular level, we use genome-wide expression profiling technologies (i.e. microarray and next-generation sequencing) to analyse spatio-temporal changes in gene expression in response to spatio-temporal targeted Tshz3 deletion experiments.
We need to perform integrative functional analyses to identify critical temporal windows and cell types that are key for ASD and/or kidney pathogenesis.
Campus / location : Luminy
Website : http://www.ibdm.univ-mrs.fr/equipe/mechanisms-of-gene-regulation-by-transcription-factors/
Team leader : Yacine Graba & Andrew Saurin
Research domain : Developmental biology
Contact : Yacine Graba - yacine.graba@univ-amu.fr ; Andrew Saurin - andrew.saurin@univ-amu.fr
The team activity is centered on the mechanisms of gene regulation by transcription factors in developmental and evolutionary processes, taking the study of Hox transcription factors as a paradigm. This is achieved by combining genomics and proteomics to set the molecular landscape, structural biology and molecular modeling to grasp the atomic details of the mechanisms, and classical molecular genetics to probe in vivo function. Past work has significantly remodeled our representation of Hox protein function, notably by the identification of the potential importance of flexible protein domain folding and plastic protein interactions for Hox transcription factor function. We believe this plasticity in interactions suits the capacity of Hox proteins to accommodate hundreds of regulatory regions and multiple protein partners, providing the molecular support for Hox protein functional diversity, specificity and evolvability.
Campus / location : Luminy
Website : http://www.ibdm.univ-mrs.fr/equipe/computational-biology/
Team leader : Bianca Habermann
Research domain : Developmental biology; Computational Biology
Contact : Bianca Habermann - bianca.habermann@univ-amu.fr
Computer-driven analysis has complemented biological research for a long time. With the beginning of sequencing and deciphering the genetic code, methods were develop that help analyze this type of data. The sequencing of parts of or entire genomes has for instance enabled us to establish a fine-tuned view on the evolution of species.
In recent years, large-scale screens and next generation sequencing is generating a tremendous amount of data, which cannot be analyzed – or understood – without the help of computational techniques. Our lab is working in computer-assisted analysis of biological data.
Our team works on two aspects in computational biology:
First, we want to make use of the vast amount of biological information available to date and put it into the perspective of biological systems in development or disease. We have chosen mitochondria to demonstrate the usefulness of large-scale data integration to understand a biological system. Mitochondria are the so-called power-houses of the cell. They provide not only energy to the cell, but are tightly involved in a multitude of metabolic functions in the cell. Their central role in cellular development and also homeostasis makes them a perfect target to investigate changes taking place in the mitochondrial system. We develop methods for the interpretation and integration of biological data and apply them to understand the – change of – function of mitochondria in a developing tissue or in a disease like Parkinson disease or cancer.
Second, we are working with protein sequence similarities in the so-called midnight zone: two related proteins have undergone so many mutations that it is difficult to detect the similarity in their sequence. We have developed methods for detecting remote homologs, remotely conserved functional domains, as well as remotely conserved orthologs – which depicts proteins that are directly evolved from each other. Currently, we are working on methods for the de novo prediction of short, functional motifs in proteins: can we, without any prior information, identify a short stretch of sequence in a protein, which performs a specific function, such as binding another protein or binding to a ligand? We are approaching this problem using either only the protein sequence or only its structure in three-dimensional space.
Campus / location : Luminy
Website : http://www.ibdm.univ-mrs.fr/equipe/genetic-control-of-heart-development/
Team leader : Robert G. Kelly
Research domain : Developmental biology
Contact : Robert G. Kelly - robert.kelly@univ-amu.fr
Our group is interested in understanding the genetic and cellular mechanisms underlying organogenesis of the four chambered mammalian heart. In particular we study an epithelial progenitor cell population known as the second heart field that gives rise to a large part of the heart by addition to the poles of the elongating heart tube in the early mouse embryo. Defects in the deployment of second heart field cells result in congenital heart defects. The second heart field is derived from a larger progenitor cell field that also gives rise to skeletal muscles of the head. Our group studies the mechanisms underlying patterning, differentiation and cell dynamics in this progenitor field, In addition, we are investigating the later development of a network of specialised conducting cell that regulate electrical activity in the ventricles.
Campus / location : Luminy
Website : http://www.ibdm.univ-mrs.fr/equipe/cellular-interactions-neurodegeneration-and-neuroplasticity/
Team leader : Lydia Kerkerian - Le Goff
Research domain : Neuroscience
Contact : Corinne Beurrier - corinne.beurrier@univ-amu.fr; Jean-Pierre Kessler - jean-pierre.kessler@univ-amu.fr
Our research activity is centered on the normal and pathological functioning of a network of brain structures called basal ganglia (BG), which plays a major role in motor control and whose dysfunction is associated with movement disorders, as in Parkinson’s disease.
Our physiological level of analysis of BG circuit function and dysfunction, which includes EEG, extracellular and intracellular electrophysiological recordings, would strongly benefit from collaboration with mathematicians to push forward signal analysis, especially when moving from single to multi-electrode/multi-unit recordings. Moreover, computational modeling of networks based on our anatomical and electrophysiological data is essential to understand BG dynamics both at structure level (e.g. local microcircuits in BG components, as the striatum, the main BG input station) and at system level (the BG network). For example, we are currently studying the physiological and pathological implications of a small population of striatal interneurons, the cholinergic interneurons, by combining optogenetics, to timely control their activity, with slice or in vivo electrophysiological recordings and behavioral approaches. These interneurons present a typical synchronized pause in their tonic activity in response to salient stimuli during reinforcement learning, pause which can be preceded or followed by bursts. We are exploring whether and how this complex pattern impacts long-term plasticity at the glutamate synapses established by the cortical inputs onto the striatal projection neurons, one main presumed cellular substrate of striatum-related learning. Iterative interactions between experiments and modeling will greatly increase our understanding of such complex operations. Computational models benchmarked based on the experimental data to be collected would be essential to dissect out the response parameters pivotal for corticostriatal long-term plasticity and its control by cholinergic interneurons. They would not only help interpreting physiological data but also predict the effect of manipulating particular components of the circuit, at cellular, network and behavioral levels, providing a test-bed for enriching the architectural and functional hypotheses prior to in vivo testing.
Our team has also long lasting interest in studying glutamate-mediated neurotransmission and the cytotoxic consequences of dysfunction at glutamate synapses, focusing on glutamate transporters. In particular, we previously provided evidence that dopamine neurons in the substantia nigra pars compacta, whose neurodegeneration is a pathological hallmark of Parkinson’s disease, show a preferential vulnerability to dysfunction of glutamate transporters.
Campus / location : Luminy
Website : http://www.ibdm.univ-mrs.fr/equipe/biology-of-ciliated-epithelia/
Team leader : Laurent Kodjabachian
Research domain : Cell and developmental biology
Contact : Laurent Kodjabachian - laurent.kodjabachian@univ-amu.fr
Ciliated epithelia harbor numerous cilia that beat in a coordinated and directional manner to propel biological fluids. They are used for locomotion by small invertebrate animals, and help airway cleaning, cerebrospinal fluid circulation in the brain, and egg transportation in the oviduct in mammals. Ciliated epithelia represent fascinating tissues to study cell fate determination, epithelial morphogenesis and regeneration, centriole and cilia biology, cell and tissue polarity, or hydrodynamics. We currently focus our attention on the mechanisms that determine the number of ciliated cells and their spatial distribution in the epithelium, and at a smaller scale those that control the number of cilia and their orientation.
We study those problems in the embryo of the amphibian Xenopus and the post-natal mouse brain, as well as in cultured ciliated cells. We strive to implement live imaging, super-resolution microscopy and genome editing in our models.
We would like to capture some of the processes we study in mathematical equations, so as to help us building up the most plausible scenarios. To reach this objective, we work hard on systematically quantifying as many biological parameters as possible.
Campus / location : Luminy
Website : http://www.ibdm.univ-mrs.fr/equipe/morphogenesis-and-cell-compartimentation/
Team leader : André Le Bivic
Research domain : Cell biology
Contact : André Le Bivic - andre.le-bivic@univ-amu.fr
Our group aims at understanding how a specialized layer of cells called epithelium has appeared during evolution to allow the development of our branch of living species ie the animals (metazoans). This was made possible with the invention of a set of proteins that promote cell-cell adhesion (E-cadherins) and the definition of the polarity axis around the adhesive structures (the polarity complexes). We want to understand at the molecular, cellular and tissue levels how these proteins interact to build a hierarchical model and to try to identify their primitive functions essential to build the first epithelial layer. In particular, how the interactions between all these protein complexes allow specific morphologies and behavior of epithelial cells by controlling a network of mechanical forces.
Campus / location : Luminy
Website : http://www.ibdm.univ-mrs.fr/equipe/cell-polarity-and-tissue-morphogenesis/
Team leader : Thomas Lecuit
Research domain : Development biology, morphogenesis, signaling, in vivo imaging
Contact : Thomas Lecuit - thomas.lecuit@univ-amu.fr
Epithelia form mechanical and chemical barriers that are both structurally robust and constantly remodelled during embryogenesis and organogenesis from nematodes to humans. Perturbations in this balance underlie solid cancer progression. How this is controlled is a fundamental unanswered question in biology. Our major ambition is to understand this problem using interdisciplinary approaches that combine the most quantitative analyses with physiological studies in whole organisms. We decipher the biochemical underpinnings of cell and tissue mechanics, namely how forces are generated and how they produce deformations, and characterize their regulation by conserved signalling pathways. Our group requires the complementary expertise of cell and developmental biologists, physicists and engineers etc.
One of the most remarkable properties of living tissues is that they combine robustness in their organisation, and extensive plasticity in their dynamics. This is especially true in epithelial sheets, where cells are usually (but not always) arranged in monolayers. Through the tight association between cells mediated by adhesion molecules, in particular E-cadherin, cells are cohesive and allow the formation of epithelial barriers that separate different physiological environments and protect the organism against pathogens. Epithelia also extensively remodel during development, and in the adult. For instance, epithelia grow and produce new cells via cell division. Cells remodel their contacts and move with respect to each other and contribute to the remodelling of the tissue.
Through these remodelling events, tissues acquire complex shapes and maintain their final organization as new cells replace dead cells.
Tissue robustness requires adhesion mediated by E-cadherin complexes. Plasticity is an active process driven by actomyosin contractility whereby cells remodel their contacts. These forces are transmitted at the cell contacts by E-cadherin complexes.
We address the following major questions:
- how forces emerge from interactions between motors, actin filaments and crosslinker.
- How E-cadherin complexes control adhesion and force transmission.
- How intercellular developmental signals control cell mechanics to drive tissue extension and invagination.
- How cell division and tissue growth affect tissue mechanics and vice versa.
We use the fruitfly Drosophila to address these problems. The major components of cell mechanics and their regulation are shared with humans and this organism lends itself to a very powerful combination of functional, molecular and physical approaches. Our group is largely interdisciplinary and employs a large battery of experimental methods ranging from biochemistry, to genetics, quantitative imaging and modelling.
Campus / location : Luminy
Website : http://www.ibdm.univ-mrs.fr/equipe/physical-approaches-to-cell-dynamics/
Team leader : Pierre-François Lenne
Research domain : Physics of living systems, imaging, morphogenesis
Contact : Pierre-François Lenne - pierre-francois.lenne@univ-amu.fr
We are interested in understanding the physical principles that underpin the morphogenesis of animals. How do cells self-organize, move and change shape to give rise to multicellular organisms,?
To tackle these questions, we study how cells interact during morphogenesis. In particular, we study the forces and the organization of supramolecular components that shape cell-cell contacts and tissues. We try also to understand how biochemical signaling and mechanics interact to organize spatially cell ensembles.
We integrate both physics (imaging/mechanics/modelling) and experimental biology to investigate morphogenetic processes in different model systems: the fruit fly, a small worm called C. elegans, and more recently, aggregates of embryonic stem cells.
We believe that we can propose a set of conceptual and experimental approaches to study living systems. We have some experience in designing and developing experiments to measure quantitative parameters, such as forces and molecular interactions, which are important to understand living systems. Our group also develops theoretical models to make quantitative and falsifiable predictions, which we test experimentally. Modeling is also a guide for new experiments. Our projects require input from physics and biology, and we are facing challenges in analysing cell behaviours, which would greatly benefit from a mathematical input.
Campus / location : Luminy
Website : http://www.ibdm.univ-mrs.fr/equipe/axon-guidance-in-the-mammalian-brain/
Team leader : Fanny Mann
Research domain : Cell and molecular biology, biochemistry
Contact : Fanny Mann - fanny.mann@univ-amu.fr
Our team is studying how neurons wire together during the development of the nervous system and how neuronal circuits are reorganized in organs affected by cancer.
Normal brain function depends on complex patterns of neuronal circuits that develop during fetal life and childhood. Several neurological disorders result from alterations that occur during the construction of brain networks.
Neurons connect to each others by extending long cables, called axons, whose growth is not random but precisely oriented towards their targets by axon guidance molecules. Our team studies the mechanisms that contribute to the fine regulation of guidance cue activities to ensure the accuracy and fidelity of axonal trajectories.
In addition to being essential to brain development, axon guidance molecules are also present in adult organisms where their expression can be reactivated under pathological conditions such as cancer. We are investigating whether their activity could contribute to the innervation of malignant tumors, a process that is still poorly characterized but can influence the course of the disease.
Campus / location : Luminy
Website : http://www.ibdm.univ-mrs.fr/equipe/neural-stem-cell-plasticity/
Team leader : Cédric Maurange
Research domain : Cell biology, neurosciences
Contact : Cédric Maurange - cedric.maurange@univ-amu.fr
We are interested in the mechanisms that regulate neural stem cell self-renewal and differentiation both during development and tumorigenesis. For this purpose, we are investigating the gene regulatory networks involved in these process and the extrinsic or cell-intrinsic signals that promote the bistable switch between self-renewal and differentiation. We are using the fruitfly Drosophila as a simple model organism that allows the use of unmatched genetic tools and the dissection of basic principles conserved in higher animals such as mammals.
In addition to the usual genetic and molecular biology tools, we would like to use the power of computer/mathematical modelling in order to rationalise our approach to specific problems.
- One way would be through the modelling of genetic networks involving different sorts of feedback loops
- Another problem we are currently particularly interested in is to decipher the rules of cellular hierarchy within tumors, a long-standing question in the cancer field.
The concept of cellular hierarchy in cancers implies that tumors are organized in a manner that is similar to tissues, with a stem cell at the top the cellular hierarchy. In tissues, stem cells give rise to intermediate progenitors that have a limited proliferation potential and rapidly differentiate in matured cells. In tumors, cancer stem cells (CSCs) may also self-renew and give rise to intermediate progenitors. However, while the balance between stem cell self-renewal and differentiation is well controlled in developing tissues allowing limited tissue growth, this balance is perturbed in tumors allowing CSCs to propagate unlimited tumor growth through unlimited self-renewal. In many cancers, we still don’t understand what dictate the choice between self-renewal and differentiation, and if we could understand this, we could design strategies to force differentiation at the expense of self-renewal, and eliminate CSCs to cure cancer.
We have established a Drosophila tumor model that contains both CSC-like cells and intermediate progenitors. We would like to collaborate with mathematicians/biophysicists to generate a computer model that may help us to understand how the choice to self-renew or differentiate is regulated in such tumors, and test predictions using the power of Drosophila genetics.
The modeling will be designed following some initial constraints that are based on some observations we have made in Drosophila neural tumors - for example:
- the 2D-3D organization of CSCs (they are organized in clusters),
- the proportion of CSCs in the tumor (constant),
- the different cell types with limited self-renewing potential they can give rise.
The models and simulations will aim to help answering the following questions:
- Is the spatial organization of the CSCs important for the regulation of the CSC population? Is the decision to self-renew or differentiate depending on the position of the CSC within the clusters and the type of contacts with the neighboring cells? What drives cluster dissemination within the tumor?
- What are the parameters that fix the proportion of CSCs?
- Which changes in parameters could explain the different CSC proportions and cluster size observed in various genetic contexts?
Campus / location : Luminy
Team leader : Alphée Michelot
Research domain : Biophysics, biochemistry, genetics and cell biology
Contact : Alphée Michelot - alphee.michelot@univ-amu.fr
Our team is working on the actin cytoskeleton, which is a network of biological polymers present in all eukaryotic cells. Cells control the dynamic assembly and disassembly of these networks, and use them as a force-generating machinery in a wide diversity of cellular processes.
We have three main objectives. Our first aim is to understand some of the basic physical and biochemical principles governing actin organization. Our second aim is to understand how cells modulate the mechanical properties of these networks thanks to a battery of molecular regulators. Our last aim is to understand how perturbations brought to the actin cytoskeleton affect various cellular functions.
Campus / location : Luminy
Website : http://www.ibdm.univ-mrs.fr/equipe/specification-of-heterogeneity-in-somatic-sensory-neurons/
Team leader : Aziz Moqrich
Research domain : Pain biology
Contact : Aziz Moqrich - aziz.moqrich@univ-amu.fr
Pain is commonly classified into acute and chronic. Acute pain arising from tissue injury is short lasting and is essential for the maintenance of our physical integrity. In contrast, chronic pain persists beyond the normal time of tissue healing and adversely impacts on our well-being. While we know a great deal about the mechanisms underlying the onset of acute pain, our knowledge on the molecular and cellular mechanisms that trigger the transition from acute to chronic pain is extremely sketchy. In the last years the Moqrich group has made two major discoveries:
1- We identified an unconventional myosin protein whose Loss-of-Function (LoF) converts a short lasting acute and reversible inflammatory, neuropathic and postoperative pain into a long lasting and irreversible chronic pain.
2- We uncovered a chemokine-like protein TAFA4 endowed with a strong analgesic effect against inflammatory and neuropathic pain conditions (Delfini et al., 2013). Therefore, using this unique mouse model in combination with state-of-the-art RNA seq and proteomics and behavioral pharmacology will allow us (i) deciphering the molecular and cellular mechanisms underlying the transition from acute to chronic pain and (ii) designing efficient preventive/curative therapeutic strategies for chronic pain.
This project fits perfectly well with the philosophy of the CenTuri project as it will require strong expertise in mouse genetics, molecular and cellular biology and Bioinformatics. A close collaboration between my group and the group of Bianca HABERMANN is already established. We believe that this close collaboration will be instrumental and will allow extending the understanding of the molecular mechanisms underlying the transition from acute to chronic pain. For example: given that chronic pain has different etiologies, we will address whether (i) chronic pain provoked by an inflammatory agent activates the same genetic programs as those leading to chronic pain after nerve injury or post-surgery, (ii) we will decipher whether inflammation-induced chronic pain markers are the same as those expressed under nerve injury or after surgery, (iii) we will also address how much contribution of the molecular changes occurring in dorsal root ganglia neurons versus those occurring in the spinal cord account for the development of chronic pain.
Campus / location : Luminy
Website : http://www.ibdm.univ-mrs.fr/equipe/immune-response-and-development-in-drosophila/
Team leader : Julien Royet
Research domain : Immunity, behavior, signalling
Contact : Julien Royet - julien.royet@univ-mrs.fr
We used Drosophila to decipher the interactions between the bacteria and its host. Our recent data suggest that in addition to trigger an immune response, infectious bacteria can modulate the behaviour of the host. We would like to identify the molecules and mechanisms that mediate the dialog between the bacteria either infectious or commensal (in the gut) and an eukaryote host.
As a teacher and future head of the Integrative Biology and Physiology Master, I am very interest to establish close contact with the person who will teach in the future CenTuri associated Master and probably to set up course that will be share by the two Masters.
As a researcher, our team is working at the intersection between microbiology, genetics, immunology and neurosciences and is therefore very into integrative research.
Campus / location : Luminy
Website : http://www.ibdm.univ-mrs.fr/equipe/muscle-dynamics/
Team leader : Frank Schnorrer
Research domain : Developmental and cell biology
Contact : Frank Schnorrer - frank.schnorrer@univ-amu.fr
We are developmental biologists that study how muscles are built.
We are particularly interested in how the molecular machines called sarcomeres, which produce the contractile forces of muscles, are built during muscle development.
We use Drosophila as our model and combine live in vivo imaging with genetics and laser micro-manipulations. We are quantifying forces at a tissue level and recently also at a molecular level to understand how forces participate in the assembly of the contractile structures.
We found that sarcomeres assemble simultaneously into long linear chains called myofibrils. We favour a force driven self-assembly mechanisms of the individual molecular components into periodic contractile units. It is important that each of this long periodic myofibrils is anchored at both of its ends in order to able to apply force. If anchoring is blocked, assembly of periodic myofibrils fails.
Institut de Neurobiologie de la Méditerranée (INMED)
Campus / location : Luminy
Website : https://www.inmed.fr/en/en-migration-neuronale-et-pathologies-du-developpement-cerebral
Team leader : Carlos Cardoso
Research domain :
Contact : Carlos Cardoso - carlos.cardoso@inserm.fr
Campus / location : Luminy
Website : https://www.inmed.fr/en/en-avenir-dynamiques-neuronales-et-fonctions-des-ganglions-de-la-base
Team leader : David Robbe
Research domain : Neureconomics, decision-making, motor control/learning, neural circuits, optimal control theory
Contact : David Robbe david.robbe@inserm.fr
The CBGCB team explores the function of a particular brain region called the striatum, which is thought to be crucial for action selection and the learning of automatisms (driving, cycling, etc.). The team examines whether the striatum's function might in fact be be to adjust the speed of our decision-making and movements based on the environment and our motivational state. This speed, also known as vigor, results from a compromise between the time required to perform our actions, which should not be too long, and the energy needed to move quickly.
In their scientific projects, the CBGCB team studies the neural circuits of the striatum that control vigor and may be responsible for behavioral differences between individuals. To this end, the team develops new behavioral tests in rodents, used theory to account for behavioral dynamics (optimal control theory, reinforcement learning) and records or manipulates the activity of striatal neurons. Better understanding the mechanisms that influence the vigor of our behaviors should help to better understand striatal dysfunctions, which are at the root of diseases such as depression, impulsive disorders, and Parkinson's disease. This could lay the groundwork for new therapies, both medicinal and behavioral, for these conditions.
Campus / location : Luminy
Website : http://www.inmed.fr/en/en-activites-precoces-dans-le-cerveau-en-developpement
Team leader : Pascale Chavis
Research domain : Neuropychiatric disorders, Circuits, Behavior, Extracellular Matrix, Cannabinoids, Sex differences
Contact : Pascale Chavis pascale.chavis@inserm.fr
Our project looks at how biological differences between males and females affect the way our brains develop, both when we’re healthy and when we have mental health problems. We do this by studying how things like genetics and our environment can change the way our brain cells communicate with each other and affect our behavior. We use advanced tools to measure both brain activity and behavior to better understand how they’re connected. Our goal is to develop new ways to help people with mental health problems by learning more about how male and female brains work differently.
Campus / location : Luminy
Website : http://www.inmed.fr/en/developpement-des-microcircuits-gabaergiques-corticaux-en
Team leader : Rosa Cossart
Research domain : Neuroscience
Contact : Rosa Cossart - rosa.cossart@inserm.fr; Arnaud Malvache - arnaud.malvache@inserm.fr
Most brain functions are encoded by specific neuronal activity patterns with characteristic temporal and spatial dynamics. In addition, there is a wide diversity of neurons often specialized in shaping a specific type of dynamics.
We study the hippocampus, a brain region supporting memory and encoding spatial and temporal information. Hippocampal dynamics result from the interaction between self-organized internal dynamics and various external inputs from the environment.
We want to know how development shapes the final functional organization of adult hippocampal networks: do they self-organize through the interaction of active neurons or do they emerge in reference to a blueprint determined by early developmental programs like genetic factors?
To describe structure-function relationships in cortical networks, we have developed a multidisciplinary approach that combines in vitro and in vivo calcium imaging of neuronal dynamics, electrophysiology, neuroanatomy, data analysis, mouse genetics and behavior.
Campus / location : Luminy
Website : http://www.inmed.fr/en/integrative-properties-of-plastic-neuronal-circuits-in-health-and-disease-en
Team leader : Valérie Crépel
Research domain : Neuronal coding, plasticity, epilepsy
Contact : Valérie Crepel - valerie.crepel@inserm.fr
The team aims at studying the synaptopathy, as well as the role of interneurons and adhesion molecules in the neuronal hypexcitability leading to the generation of seizures in epileptic conditions. Our studies are conducted at several scales: from the subcellular compartment to the neural microcircuit. The research group has a long history in the study of the physiopathology of Temporal Lobe Epilepsy (TLE), which is one of the most common forms of partial epilepsy in adults. In the epileptic hippocampus, dentate granule cells (DGCs) form new recurrent synapses. The team discovered that these synapses are not only aberrant in their localization, but they also are operating via a subtype of glutamatergic receptors not present in naïve conditions, the kainate receptors (KAR, Epsztein et al., 2005).
For the past ten years, our studies tackle the impact of these ectopic KARs in neuronal coding and seizure generation in DGCs from animal models of TLE. We showed that ectopic KARs, in addition to a reorganisation of the network, play a central role in TLE by deeply altering the neuronal coding properties, leading to the generation of pathological activities including seizures (Epsztein et al., 2010; Artinian et al., 2011, 2015; Peret et al., 2014). Furthermore, we demonstrated that the expression of GluK2/GluK5 subunits in these ectopic kainate receptors is instrumental for the generation of seizures (Peret, Christie et al., 2014; Crepel & Mulle, 2014). Based on these data, the team published a patent (INSERM Transfer, WO2015036618-A1/N° 10,016,424), and is currently involved in the maturation and the development of new antiepileptic approaches targeting these ectopic kainate receptors.
In this context, the team is a member of the FHU EpiNext.
In parallel, the research group is focusing on the role of some cell adhesion molecules (CAMs) associated with Kv1 potassium channels, in neuropathies characterized by the generation of seizures. More specifically, we study the involvement of the CAMs Caspr2 (CNTNAP2) and LGI1 in neurological diseases of autoimmune or genetic origin leading to epilepsy (Canali et al., 2018; Pinatel et al., 2015; Hivert et al., 2019). We decipher the targeting and the assembly of the Kv1 complex, particularly at the axon initial segment and nodes of Ranvier, in physiological and pathological conditions (Pinatel et al., 2017; Brivio et al., 2017; Hivert et al., 2016).
The FHU DHUNE approved this research program Scientific Interests. The team tackles three major topics:
1)The role of kainate receptors in seizure activities
2)The role of principal cells and interneuronsin the alterations of coding properties and the generation of seizures in epileptic conditions
3)The role of cell adhesion molecules involved in neuropathies and seizures Team’s skills: In vitro and in vivoelectrophysiology (local field potential, patch-clamp), calcium imaging, cell biology, cell cultures, behaviorand virtual reality, rodent models.
Campus / location : Luminy
Website : http://www.inmed.fr/en/neuronal-coding-of-space-and-memory
Team leader : Jérôme Epsztein
Research domain : Neurosciences
Contact : Jérôme Epsztein - jerome.epsztein@inserm.fr
Where are we? Where do we want to go and how do we get there? Our research group is interested in how spatial information is represented in the brain, stored and used for efficient navigation. How do external (from the outside world) and internal (linked to our own movements) information combine to generate a cognitive map of our environment? How is our position and direction coded and constantly updated to navigate this map?What is the role of synaptic inputs and intrinsic neural properties in this process? We address these questions at the behavioral, circuit and single cell levels.
Campus / location : Luminy
Website : https://www.inmed.fr/en/neural-computation-and-dynamics
Team leader : Lorenzo Fontolan
Research domain : decision making, motor control, neural networks, attractor dynamics, memory
Contact : Lorenzo Fontolan lorenzo.fontolan@inserm.fr
To foster survival in a dynamic world, animals must be able to quickly adapt their behavior in response to unpredictable environments. The nervous system has the ability to assign the appropriate responses to sensory stimuli according to its internal state (e.g. the subjective perception of time, emotional states) or an external context (e.g. environmental demands, social conventions). For example, when you are at the stadium attending a game and happen to see an old friend a few seats away, you will not hesitate to say “Hello!” with a loud greeting. Instead, if the fortuitous encounter happened at a library, the same greeting would be barely whispered so not to disturb readers.
The research goal of the Neural Computation and Dynamics lab is to understand the neural basis of flexible behavior by developing computational models of brain circuits. To study the synaptic and circuit mechanisms that underlie flexible behavior we employ theoretical and numerical techniques from dynamical systems, physics, and machine learning. We collaborate with experimental labs around the world to draw inspiration from their work and to test our models.
Campus / location : Luminy
Website : http://www.inmed.fr/en/en-activites-precoces-dans-le-cerveau-en-developpement
Team leader : Rustem Khazipov
Research domain : Neurophysiology, developmental neurosciences
Contact : Rustem Khazipov - roustem.khazipov@inserm.fr
The objective of our team is to understand the structural and functional development of the brain, and more particularly cortical activity, under physiological and pathophysiological conditions. Our investigations in rodent models but also in human patients, through collaborations with clinicians, aim at understanding the early activity patterns involved in brain development. Through a multidisciplinary approach combining brain activity recording, cellular and molecular analyses, and behavioral investigations, we focus on GABAergic neurotransmission and its dichotomy of action through development, and several neurodevelopmental pathologies including brain hypoxia, trauma, autism spectrum disorders, intellectual disability, and epilepsy.
Campus / location : Luminy
Website : http://www.inmed.fr/en/neurodevelopment-and-prader-willi-syndrome
Team leader : Françoise Muscatelli-Bossy
Research domain : Neurosciences, development
Contact : Françoise Muscatelli - francoise.muscatelli@inserm.fr; Valéry Matarazzo - valery.matarazzo@inserm.fr
Our team is interested in understanding how two imprinted genes belonging to the same family of MAGE genes are involved in brain development and behavioural processes which are found altered in Prader-Willi and autism. We are at the interface between genetic and epigenetic regulations that are involved in brain control for postnatal life. Our objectives
- Decipher the development of the brain oxytocin (OT)-network. In the context of centuri, we aim to modelize the set-up of this network and the key stages of development in normal and pathological conditions.
- Decipher the interplay and genomic/transcriptional regulation of MAGE genes. In the context of century we aim to use bioinformatic tools to analyse the MAGE network in mammmals . Collaborations are already ongoing with TAGC.
The team is significantly involved in Training/Education of Neurobiology at the Master levels (Master of Neurosciences and DI), at the Engineer School Polytech Biotech (Luminy) and in 2 Amidex PhD programs (ICN Neurosciences, and Biotrail).
Campus / location : Luminy
Website : www.ranczlab.com
Team leader : Ede Rancz
Research domain : Neuroscience
Contact : ede.rancz@inserm.fr
Understanding the brain requires hypotheses about what it is computing. Our laboratory adopts the predictive coding framework, which proposes that internal, generative models constantly attempt to predict incoming sensory input. The biology behind internal models, how they emerge, and a mechanistic understanding of their functioning is at the core of our research programme.
We work on the mouse visual cortices, especially layer 5 pyramidal neurons, that convey the main output of cortical computations. We study the inputs they receive on their dendrites, the computations they can do, and the effect of their activity on eye-movements. We are using a combination of techniques, e.g., in vivo recordings on a purpose-built apparatus combining visual virtual reality with animal-driven physical rotation, corresponding to sensory inputs from the visual and vestibular senses. This way we can introduce prediction errors through sensory mismatch to interrogate the enigmatic internal models.
Institut de Neurosciences des Systèmes (INS)
Campus / location : Timone
Website : https://ins-amu.fr/tng
Research domain : Neuroscience
Contact : Viktor Jirsa - Viktor.JIRSA@univ-amu.fr
The scientific objective of TNG is to uncover the mechanisms underlying the emergence of brain function from the spatiotemporal organization of large-scale brain networks. Our focus is indeed brain network dynamics. The dynamics of brain networks on the large scale has certain characteristics, typically less relevant in traditional neural network studies. These large-scale features comprise the presence of multiple spatial and temporal scales, time delays via signal transmission, and a highly non-trivial (i.e. inhomogeneous and anisotropic, thus translationally variant) connectivity. Together, these factors impose constraints upon the network dynamics, which is linked to the emergence of behavioral function/dysfunction and can be imaged in the human brain non-invasively (fMRI, EEG, MEG) and invasively (sEEG, iEEG). All of our work in TNG can be understood within this framework and comprises theoretical and computational studies of large-scale network behavior (resting state fluctuations, stimulated brain dynamics, self-organized routing), the development of neuroinformatics tools for studying brain networks (both simulated and empirical) up to the whole-brain scale, and its applications to concrete functions (such as hand writing), dysfunctions (epilepsy, stroke) and aging.
Over the years, TNG has developed a reputation as a long-standing contributor and pioneer in the field of large-scale brain network dynamics, linking generative brain network models to human brain imaging data. Key to the success has been the tight interaction of mathematics, computation and experiment, in which mathematics often needed to abandon “rigor” and experimental data were often looked at “non-biologically” from the system perspective. This unconventional approach contributed to creating a new field, brain connectivity, which shows an uninterrupted series of 15 annual Brain Connectivity workshops and the creation of a new journal with the same name.
Currently ongoing research projects in TNG include
- The Virtual Brain neuroinformatics project
- Large-scale brain network theory
- Brain Dysfunction (Epilepsy)
See below for more details on each of these pillars of research.
Institut de Neurosciences de la Timone (INT)
Campus / location : Timone
Website : : https://brainets.github.io/
Team Leader: : Andrea Brovelli and Emmanuel Dauce
Research domain : Neuroscience
Contact : Andrea Brovelli and Emmanuel Dauce
Research domain : Neuroscience
BraiNets is a research team at the interface between cognitive and computational neuroscience composed by Andrea Brovelli (DR2, CNRS) and Emmanuel Daucé (MC, Ecole Centrale Marseille). BraiNets exploits most advanced theoretical approaches and computational tools from artificial intelligence and statistics to address open questions in cognitive and system neuroscience. In particular, the main objective of Brainets' research is to better understand both the neural and computational bases of learning. At the neural level, we aim at clarifying the role of cortico-cortical interactions in learning and goal-directed behaviors. At the computational level, we seek to develop innovative computational models inspired from artificial intelligence and anchored on principles of biological systems. Our objective is to map the predicted learning computations onto cortico-cortical interactions and brain network dynamics. The main theoretical approaches and methodology characterizing BraiNets are: i) Functional Connectivity analysis and information theory; ii) computational neurophysiological and analysis of brain network data (MEG, intracranial EEG, LFP and fMRI); iii) Bayesian probabilistic modeling and reinforcement learning; iv) neural networks models and neurocomputational modeling; v) machine learning
Campus / location : Timone
Website : https://conect-int.github.io/
Team Leader: Laurent Perrinet
Research domain : Neuroscience
Contact : Laurent Perrinet - laurent.perrinet@univ-amu.fr
Neuroscience has experienced a revolution over the past decade, during which tremendous technological advances across several disciplines have dramatically expanded the frontiers of experimentally accessible neuroscientific facts. It is an evidence that the close collaboration between experimentalists and theoreticians in neuroscience is essential to develop mechanistic as well as quantitative understandings of how the brain performs its functions. However, for such collaborations to be effective, experimentalists must be well aware of the approaches and challenges in modeling while theoreticians must be well acquainted with the experimental techniques, their power and the challenges they present. The CONECT team is a center within the Institut de Neurosciences de la Timone which aims at structuring of these different components through a center independent of existing teams. CONECT has also the ambition to contribute to the training of a new generation of neuroscientists who will have all these qualities. CONECT aims to serve as an incubator within INT to promote the use of methodologies developed in computational and theoretical neuroscience, a catalyst for the creation and maturation of new multi-approach projects within INT and the local community.
Institut des Sciences du Mouvement (ISM)
Campus / location : Hôpital Sainte-Marguerite
Website : https://ism.univ-amu.fr/en/en/BMI
Team Leader: Jean-Louis Milan
Research domain : Biomechanics and Bioingeneering
Contact : Jean-Louis Milan - jean-louis.MILAN@univ-amu.fr
Our group develops in silico mechanobiological models of cells and tissues.
At the cellular level, our modeling reproduces the dynamics of the cytoskeleton to analyze how cells mechanically adapt to their environment.
For example, we have studied in silico the mechanism of curvotaxis, or how cells migrate as a function of substrate curvature.
Our cellular model reproduces the cell migration phenomena observed experimentally on curved substrates. In addition, it provides a mechanical explanation involving the tension of the nucleus and cytoskeleton and the decentering of the nucleus towards concave areas. This off-centering of the nucleus, like a ball and chain, induces a bias in migration. We propose to extrapolate the scope of this cellular model of curvotaxis to the case of changing and dynamic cellular environments such as those encountered in vivo during embroyogenesis. Our approach may also help in the design of intelligent deformable substrates to guide cell migration as a new strategy in tissue engineering.
We are also developing in silico models of tissue formation and differentiation as a function of mechanical stress.
Laboratoire d'Ingénierie des Systèmes Macromoléculaires (LISM)
Campus / location : Luminy
Website : https://hpi.afmb.univ-mrs.fr/
Team leader : Alain Roussel
Research domain :Microbiology, immunology, virology, molecular modelling
Contact : Alain Roussel - alain.roussel@afmb.univ-mrs.fr ; Isabelle Imbert - Isabelle.IMBERT@univ-amu.fr
STUDYING HOST-PATHOGEN INTERACTIONS AT ALL SCALES
Our team is interested in understanding and dissecting interactions of pathogens with their hosts. Our targets of interest are diverse and include: (i) bacteriophages and human RNA viruses, (ii) type 6 and type 9 secretion systems, and (iii) the host immune response in model organisms such as Drosophila and C. elegans worms, with a focus on antibodies. Understanding host-pathogen interactions is at the heart of the fight against pathogens involved in infectious diseases (viruses, bacteria).
Hence, one of our research interest is the replicative machine of RNA viruses. RNA viruses are responsible for all emerging infectious diseases (Ebola, Zika, Dengue, Coronaviruses…). Unfortunately, most of the time, we have few weapons to fight them, due to a certain lack of knowledge of their propagation cycle.
By combining multidisciplinary approaches such as biochemistry, enzymology, structural biology on one hand and computing science and artificial intelligence methods on the other hand, we aim to dissect fascinating RNA virus replication machineries. These data will lay the groundwork for the discovery of anti-viral molecules. Indeed, viral replicases have already proved their worth with, for example, the development of therapies against HIV and HCV.
In silico viral RNA polymerization modelling
Modelling of complex biological systems is a growing field of research and a valuable tool for public health. Examples of applications include modelling the dynamics of virus transmission, predicting circulating strains in vaccine manufacturing or modelling the occurrence of resistance mutations after treatment with antivirals.
We develop an integrative system using artificial intelligence approaches to anticipate the biodiversity of RNA virus polymerases. Our strategy relies on the use of semantic technologies (especially ontology engineering) associated to in silico simulation. Then, an iterative process is engaged where in silico results are confronted with the in vitro experimentations.
Laboratoire de Chimie Bactérienne (LCB)
Campus / location : Joseph Aiguier
Website : http://lcb.cnrs-mrs.fr/spip.php?article947
Team leader : Emanuele Biondi
Contact : Emanuele Biondi - ebiondi@imm.cnrs.fr
Alphaproteobacteria is a class of gram negatives with remarkable characteristics. They often interact with or infect plants and animals. An alphaproteobacterium was the ancestor of mitochondria and a bacterium of this class is the most abundant species in the oceans. Moreover alphaproteobacteria often undergo to cellular differentiation and present a regulation of cell cycle (coordination between DNA replication, cell division and cellular differentiation) with same points of contact with eukaryotes. In the team Biondi, we aim to understand logic and principles of cell cycle regulation and asymmetrical cell division in two well-known model systems, Caulobacter crescentus and Sinorhizobium meliloti (see figure).
In Caulobacter crescentus, CtrA (response regulator of Two-Component systems) controls many important functions (motility, DNA methylation, cell division, chemotaxis, stalk and pili biogenesis) and blocks directly the origin of DNA replication. In our lab we investigate the mechanisms of CtrA phosphorylation by CckA and ChpT (Biondi et al., 2006, Nature) which is blocked by the DivJ-(PleC)-DivK module and c-di-GMP. We also investigate the regulation of transcription by CcrM-dependent genome methylation and GcrA (Fioravanti et al., 2013, PLOS Genetics). In Sinorhizobium CtrA also controls cell cycle but it also involved in nitrogen-fixing bacteroids differentiation (Pini et al., 2013, Mol Micro ; Pini et al., 2015, PLOS Genetics).
Campus / location : Joseph Aiguier
Website : http://lcb.cnrs-mrs.fr/spip.php?article983
Team leader : Axel Magalon
Contact : Axel Magalon - magalon@imm.cnrs.fr
One of the most fundamental challenges of bacterial cell metabolism is to ensure a reliable balance of carbon, electrons and energy in the highly variable biological environment. In this context, bacterial respiration is essential as it provides energy in the form of ATP and of an electrochemical gradient generated across the membrane which powers transport of molecules (proteins, ions and antibiotics) or cell motility.
Our focus on anaerobic bacterial respiration is stimulated by its emerging role in human microbiota and its impact on health. Indeed, inflammation of the gastrointestinal and respiratory tracts is associated with the bloom of facultative anaerobic γ-proteobacteria. Most respiratory complexes using alternative substrates to O2 are molybdoenzymes belonging to an ancient protein family found in most prokaryotes.
Using multi-scale approaches ranging from atomistic investigation of molecular processes to cell biology studies, our team studies (i) how to get a fully active respiratory complex in the cell, (ii) what determines reactivity of respiratory complexes toward their substrates and (iii) how optimization of respiration is made in response to fluctuating environmental conditions. To this end, collaborations are settled with laboratories working in various fields such as chemistry, physics, crystallography, genetics, microscopy and medicine as interdisciplinarity is key to investigate complex biological systems.
In the recent years, we have :
- identified and provided a comprehensive understanding of a new dedicated chaperone involved in the activation of formate dehydrogenases (Thome 2012 JBC, Arnoux 2015 Nat com)(Figure 1),
- provided unprecedented structural details about the mode of interaction of quinones with respiratory complexes (Arias-Cartin 2010 JACS, Grimaldi 2012 JBC, Rendon 2015 BBA),
- unveiled a new function of lipid binding to respiratory complexes (Arias-Cartin 2011 PNAS),
- demonstrated that dynamic subcellular localization of respiratory complexes is a critical factor in the control of bacterial respiration (Alberge 2015 eLife)(Figure 2).

Figure 2 : How optimization of respiration is made in response to fluctuating environmental conditions ? Nitrate reductase is a major respiratory complex under anaerobiosis in most γ-proteobacteria including Escherichia coli. We showed that its localization is submitted to tight spatiotemporal regulation in response to metabolic conditions.
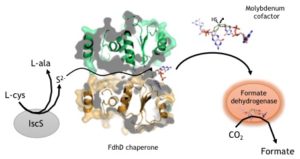
Figure 1 : How to get a fully active respiratory complex in the cell ? Production of active formate dehydrogenases relies on the activity of a dedicated chaperone ensuring sulfur atom transfer from IscS to the molybdenum cofactor prior its subsequent delivery to the enzyme.
Campus / location : Joseph Aiguier
Website : https://sites.google.com/site/myxolab/research
Team leader : Tâm Mignot
Contact : Tâm Mignot - tmignot@imm.cnrs.fr
Our team studies how cells make decisions to assemble multicellular structures. Specifically, we use Myxococcus xanthus a social bacterium which uses collective surface motility to predate and form fruiting bodies in hostile environments. In this model system, we have shown that bacteria move using a novel molecular motor that assembles at the bacterial cell pole to form so-called bacterial focal adhesions complexes. We have also studied how motility is regulated by environmental cues and shown that complex signaling networks converge into the spatial regulation of the motility complex, controlling its spatial assembly at the cell pole. Using single cell and computational approaches, we are now investigating how these regulations translate to the formation of multicellular patterns. The long-term goal is to build a high-resolution model of the cell-cell regulations that underlie the formation of bacterial multicellular structures.
Campus / location : Joseph Aiguier
Website : http://lcb.cnrs-mrs.fr/spip.php?article946
Team leader : Béatrice Py
Contact : Béatrice Py - py@imm.cnrs.fr
In the last 15 years, the team has worked on stress responses. Stress means “unbalanced” and for a bacterium, to live under stress is (almost) a routine way of living. Most bacteria are constantly exposed to fluctuating environments and what we are interested in is how they sense, react and adapt to get back to homeostasis. Our research has dealt with general basic processes, all controlled by ubiquitous and conserved molecular devices (respiration, iron and Fe-S biology, protein oxidation and repair, antibiotic resistance) using E. coli as a model or Salmonella, a human pathogen. Recent project are orientated towards understanding antibiotic mode of action and how this could eventually help in fighting against resistance.
Campus / location : Joseph Aiguier
Website : http://lcb.cnrs-mrs.fr/spip.php?article1008
Team leader : Long-Fei Wu
Contact : Long-Fei Wu - wu@imm.cnrs.fr
Our research interest is to understand synthesis, function and ecological implication of bacterial motility apparatus.
Magnetotactic bacteria (MTB) are a phylogenetic, physiological and morphological divers group of Gram-negative bacteria. They display a myriad of cellular morphologies, including coccoid-ovoid, rod-shaped, vibrioid, spirilloid and, the most remarkable multicellular forms referred to as multicellular magnetotactic prokaryotes (MMP or magnetoglobules in short). They have the unique capacity of aligning and swimming along the geomagnetic field lines, a behavior referred to as magnetotaxis. To better understand the magnetotactic mechanism we have studied the synthesis and function of flagellar apparatus of magnetotactic bacteria.
Magnetospirillum magneticum strain AMB-1 has a flagellum at each pole of their spiral-shaped body (amphitrichously flagellated). Using fluorescence labeling, single-cell tracking and swimming pattern analysis we found that asymmetric rotation of the flagellum counter-clockwise (CCW) at the lagging end and clockwise (CW) at leading end propel the magnetospirillum AMB-1. Simultaneous change of the rotation directions leads to reversal of the swimming direction while symmetric rotation of the two flagella (either CCW or CW) results in tumble of the cell. By biochemical analysis, we showed that the flagellin of AMB-1 is glycosylated. Near the flagellin gene, several putative glycosyltransferases genes were found. Among these genes we have identified a gene encoding for a Maf (Motility Accessory Factor) homologue essentially found in pathogenic bacteria genera (like Campylobacter, Helicobacter, Legionella…) and we have studied its role and its structure. We have already showed that, in a Δmaf mutant, the flagellin is still produced but not glycosylated and the bacteria are unflagellated. The structure of Maf presents three different domains. The central domain displays close structural similarity with the structure of a sialyltransferase from C. jejuni.
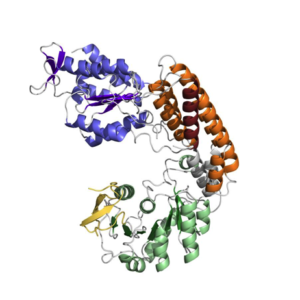
Fig 1 : The structure of Maf is organized into three domains : a N-terminal domain of unknown function (blue), a central domain with a modified sialyltransferases fold (green and yellow) and a C-terminal exhibiting resemblance with flagellin export chaperones and flagellins (orange)
The magneto-ovoid strain MO-1 that has two flagellar bundles on one side of the cell (bilophitrichously flagellated). In collaboration with Prof. K. Namba’s laboratory at Osaka University we have discovered the most exquisite architecture of the MO-1 flagellar apparatus. Seven flagella filaments (composed of 12 kinds of glycosylated flagellins), and 24 fibrils are arranged in seven intertwined hexagonal arrays enveloped within a sheath (Fig. 2). Such a robust propeller probably allows chemo-litho-autotrophic bacterium MO-1 swimming across chemically stratified layers in marine sediments to obtain O2(electron acceptor, on surface), reduced inorganic sulfur compound (electron donors, in deep sediment) and bicarbonate for its growth.
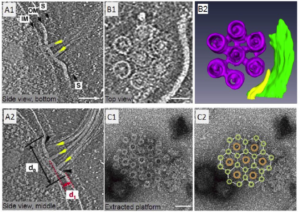
Fig. 2 Locomotion apparatus of the strain MO-1. Panels A1 and A2 are two-tomograph slices showing side view of a shallow bowl-like platform with a diameter of 230 nm (d0) and a depth of 46 nm (d1) and flagella with yellow arrow heads. Panels B1 and B2 are cryo-eletron micrograph and re-constituted image of flagella array. Panels C1 and C2 show the extracted platform with 7 flagella (brown) and 24 fibrils (light green). Scale bar = 100 nm. Ruan et al (2012). Proc. Natl. Acad. Sci. USA. 109 : 20643-8..
The invention of multicellularity is one of the principal thresholds in evolutionary history. To shed light on the origin, evolution and function of multicellularity we perform ecological, microscopic, genomic and biophysical studies of magnetoglobules. This project is carried out in collaboration with Prof. T. Xiao’s laboratory at Institute of Oceanology of
Chinese Academy of Sciences,
Magnetoglobules were first discovered in the Rodrigo de Freitas lagoon in Brazil, and then observed worldwide. Typically 10–40 cells arrange in spherical or mulberry-like multicellular entity (Fig 3, A). Besides the mulberry-like, we have first observed at the Mediterranean Sea and then collected from both the Mediterranean Sea and the China Sea another group of magnetoglobules : the ellipsoid or pineapple-like MMPs (Fig. 3 up, Panel B). They are composed of about 40 phylogenetic-identical cells arranged in 4–6 interlaced circles. The spherical and ellipsoidal magnetoglobules belong to different genera of delta-Proteobacteria and co-occur in the sediments at the same sampling sites with one or the other as the dominant morphotype. They exhibit a specific ping-pong motility which involves a rapid backward movement and a slower forward movement along magnetic field lines. How these cells coordinate their surface flagellar rotation and what is the ecological implication of the ping-pong motion are enigmas.
Institut Méditerranéen d'Océanologie (MIO)
Campus / location : Luminy
Website : www.oceanbridges.net
Team leader: Mar Benavides
Research domain: Microbial Oceanography
Contact: Mar Benavides - mar.benavides@ird.fr
Marine microbes are embedded in moving volumes of seawater, which change in chemical and physical properties as they travel the ocean. These interactions occur across dynamic spatiotemporal scales and need to be studied with a multi-scale and transdisciplinary approach.
Our goal is to bridge the spatiotemporal scales at which microbes and resources interact in the ocean. On seagoing expeditions and culture experiments in the lab, from nanometers/seconds to the global ocean/years: we combine multiomics, advanced microscopy, single-cell mass spectrometry, geochemistry, satellite imagery and modeling to unveil how microbes shape nutrient cycles in the ocean.
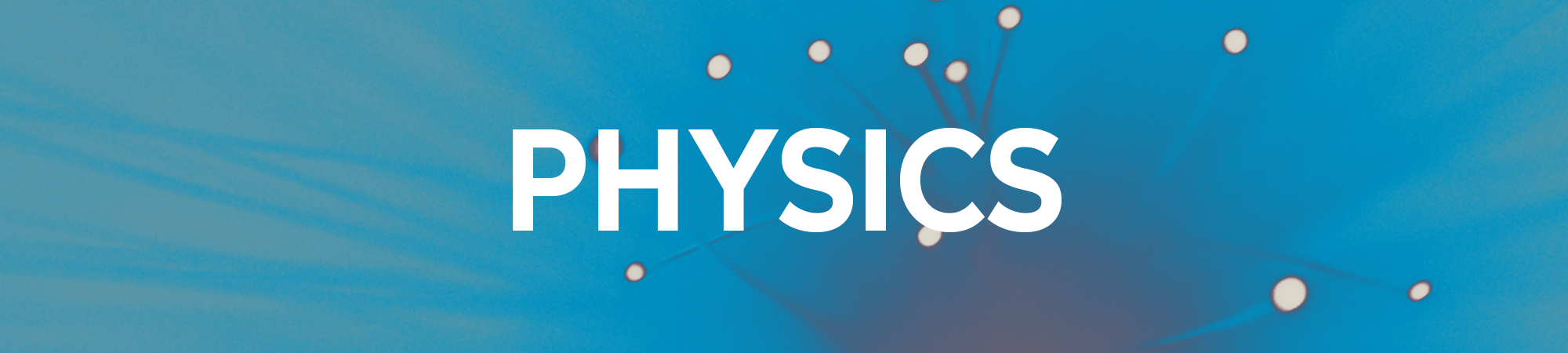
Centre Interdisciplinaire de Nanoscience de Marseille (CINaM)
Campus / location : Luminy
Website : http://www.cinam.univ-mrs.fr/cinam/team/physique-et-nano-micro-ingenierie-pour-le-vivant/
Team leader : Anne Charrier
Research domain : Biophysics, in vitro approach, cell mechanics and dynamics
Contact : 06 62 92 28 35 / anne.charrier@univ-mrs.fr
Our research is divided into two main topics. The first one deals with the development of biomimetic approaches that combines microfluidic and optical microscopy to decipher biological processes. We are interested in understanding the mechanisms of red blood cell (RBC) spleen filtration in general and investigate the mechanisms of extreme cell deformability and the role of active processes such as the role of mechano-sensitive ion channels. In another project, we investigate the mechanisms involved in the filtering of RBCs with vacuoles in splenectomized patients combining mechanical and bio-chemical processes.
Our second topic deals with wood morphogenesis and aims specifically at understanding the mechanisms involved in wood reorientation. We gather knowledge on the mechanical behavior of the cell wall layers, of their interactions and of their stress state. We explore how tension is generated at the molecular level and how this tension is transferred between the different parietal layers to induce reorientation at the micro-scale.
Campus / location : Luminy
Website : https://www.cinam.univ-mrs.fr/cinam/le-centre/annuaire/fiche-personnel/?idu=730
Team leader : Emily Gehrels
Research domain : Biological physics, tissue dynamics, cell fluctuations, non-equilibrium effects
Contact : emily.GEHRELS@univ-amu.fr
During embryonic development, tissues flow and fold to create the complex form required to support life. For tissues to maintain integrity while undergoing large flows, the composing cells need to dynamically rearrange to allow the tissue to act as a fluid instead of a solid. It has been proposed that dynamic fluctuations in cell-shape could facilitate cell rearrangement and therefore fluidization of the tissue, but there is a lack of experimental evidence to support or refute this hypothesis. Moreover, is unknown if such fluctuations would arise from passive thermal effects or from active, energy-consuming processes in the cells.
Our research utilizes live-imaging of Drosophila embryos to quantify cellular dynamics and correlate them to tissue flow. We combine techniques from biology and physics to (1) examine the role that cell-scale fluctuations play in tissue fluidization, (2) measure how these fluctuations depend on energy consumption within the cell, and (3) uncover whether changes in tissue fluidization result from thermal effects, or from non-equilibrium (energy-consuming) processes. To quantify and interpret the results from our experiments, we relate them to biophysical models of tissue mechanics/ dynamics and to non-equilibrium statistical mechanics of living systems.
Campus / location : Luminy
Website : http://www.cinam.univ-mrs.fr/cinam/en/the-lab/directory/user-profile/?idu=412
Team leader : Emmanuèle Helfer
Research domain :Biological physics, in vitro approach, cell mechanics and dynamics, pathologies, actin-based processes, membrane physics
Contact : Emmanuèle Helfer - helfer@cinam.univ-mrs.fr
We are interested in biomimetic approaches combining microfluidics and microscopy to decipher biological processes, from the subcellular scale to the multicellular scale.
At the subcellular scale, we reconstitute in vitro actin-based processes with minimal systems (synthetic membranes, purified proteins) in order to control physical and biochemical parameters. From mesoscopic observations (with optical microscopy techniques) we derive molecular interplays and self-assembly dynamics (protein-protein, protein-membrane interactions). We are currently reconstituting a minimal adhesion complex, in collaboration with K. Sengupta (CINaM, CENTURI) and external groups (Gif-sur-Yvette, Bordeaux), to decipher the relationship between focal adhesion regulation and acto-myosin force. Another project aims at investigating the role of the actin cytoskeleton in intracellular endosomal trafficking, more precisely how actin contributes to membrane/protein partitioning at the endosomal membrane.
At the cellular/multicellular scale, we investigate the dynamics of cells under mechanical constraint, in healthy and pathological conditions. A first project, in collaboration with A. Viallat (CINaM, CENTURI), is dedicated to deciphering the physical mechanisms of red blood cell splenic filtration, using innovative microfluidic devices. A second project, joint with a biologist in Marseille, recently started which aims at understanding the senescence mechanism related to nuclear envelope defects, in the frame of premature aging syndromes. From studies on single cells we plan to develop with JF. Rupprecht (CPT, CENTURI) a physical model of nucleus rheological properties. In parallel we investigate the mechanotransductional response of cell assemblies to mechanical stresses. We expect our results will lead to an understanding of the pathway to atherosclerosis.
Campus / location : Luminy
Website : https://www.cinam.univ-mrs.fr/cinam/en/the-lab/directory/user-profile/?idu=722
Team leader : Nicolas Levernier
Research domain : Stochastic dynamics, biophysics, statistical mechanics
Contact : Nicolas Levernier - nicolas.LEVERNIER@univ-amu.fr
In recent years, interest in out-of-equilibrium systems has been booming. Indeed, while they can be found everywhere from the molecular scale to that of living organisms or climatic systems, in physics, biology or even humanities, these systems have properties that are quite different from those at thermal equilibrium. One of the current major theoretical challenges is to extend the concepts and laws of thermodynamics and statistical physics to non-equilibrium systems. While some progress has been made in this direction, it mainly concerns stationary thermodynamic observables. Very little is known about the influence of out-of-equilibrium phenomena on kinetics.
I am interested in the theoretical study of stochastic kinetics for complex system far from equilibirum, mainly with tools of stochastic calculus. Also the methods I am developping is expected to be robust to the precise context, a particular focus is done on biological questions such as pathogen capture, T-cell activation, bacterial infection or nucleation during liquid-liquid phase separation.
Campus / location : Luminy
Website : https://www.pierre-ronceray.net/
Team leader : Pierre Ronceray
Research domain : Modeling and Measuring Soft Living Matter
Contact : Pierre Ronceray - pierre.ronceray@univ-amu.fr
Understanding the organization and dynamics of living matter, from the protein level to the tissue level, is a tremendous challenge from a physicist’s point of view. My goal is to identify simple physical laws governing this complex, heterogeneous, non-linear, out-of-equilibrium state of matter. I propose a dual theoretical approach to this problem. On the one hand, I address the direct problem: through analytic calculations, computational modeling and collaborations with experimentalists, I explore the assembly, mechanics and thermodynamics of cellular structures. On the other hand, I design methods for the inverse problem: inferring the dynamical properties of these systems – such as force, diffusion and stress fields – from microscopy data. Indeed, while these quantities are central objects in physical theories of soft biological matter, they are not directly observable: to advance our understanding of biological matter, novel data analysis methods adapted to modern experimental techniques are required.
Campus / location : Luminy
Website : http://www.cinam.univ-mrs.fr/cinam/en/the-lab/directory/user-profile/?idu=184
Team leader : Kheya Sengupta
Research domain : Biophysics of membranes, cell adhesion/mechanics
Contact : Kheya Sengupta - sengupta@cinam.univ-mrs.fr
Our current research is focused on adhesion of living and model cells. The adhesion of cells is a fundamental biological process with implications for functions ranging from tissue morphogenesis to immune response. Very often, the primary focus in cell adhesion research is on identifying the relevant adhesion proteins and signaling pathways. However, for a complete description, it is essential to also understand the physics underlying the processes that govern the adhesion. In order to study a complex phenomenon like cell adhesion, in-vitro experiments using cell mimetic systems are often useful since the experimental conditions can be better controlled. Moreover, the simplicity of such systems facilitates theoretical modeling. The insight gained from such bio-mimetic studies can then be applied to real cells. In our research, on one hand we try to make realistic models of adhering cells using giant unilamellar vesicles/supported lipid bilayers and on the other hand we study the adhesion and activation of T lymphocytes under biomimetic conditions, hoping to ultimately link the insights gained from model systems to cellular observations.
In particular, our current interests include:
- Development of new protocols for Nano-patterning of biomimetic and soft substrates. Collaboration: Anne Charrier (CINaM/CENTURI).
- Fluctuations and adhesion of lipid membranes. Collaboration: Rudolf Merkel (Forschungszentrum Julich), Ana Smith, (University of Erlangen-Nuemberg), and Udo Seifert, (University of Stuttgart, Germany). Emerging collaboration with Emmanuelle Helfer (CINaM/CENTURI), Christophe Le Clainche (I2BC, Paris), Gregory Giannone (IINA, Bordeaux)
- Structure and dynamics of the immune-synapse. Collaboration: Laurent Limozin, (LAI/CENTURI), emerging collaboration with Pierre-Henri Puech (LAI/CENTURI) Bernard Malissen (CIML/CENTURI), and Jacques Nunes (CRCM/IPC). In the immediate future, I hope to extend my collaboration with immunologist and to go deeper into the biophysics of immune cells. I also hope to interact with other biologists, especially in application of model membranes, I expect fruitful exchanges with both biologists and theorists around concepts emerging from my model membrane studies.
Campus / location : Luminy
Website : http://www.cinam.univ-mrs.fr/cinam/en/the-lab/directory/user-profile/?idu=424
Team leader : Annie Viallat
Research domain : Biological physics, cell rheology, fluid and elasticity
Contact : Annie Viallat - viallat@cinam.univ-mrs.fr
Today, our interests are on active matter, applied to the dynamics of complex biological fluids (muco-ciliary dynamics), blood cells and vesicles (coupling between fluid and deformable objects) and biomimetic tools (microcapsules with innovative mechanical or encapsulation properties, physiological micro-flows created in specific microfluidic devices, devices for measuring cellular migration in 3D). We are particularly interested in fluid-elasticity coupling in living matter.
Our main projects are
- to understand the dynamics of blood cells in concentrated suspension (blood) in networks mimicking blood capillaries. The aim is to describe cell aggregation and vaso-occlusion as a function of the cellular mechanical properties (adhesiveness and deformability), in particular for genetic diseases such as sickle cell anemia and hereditary spherocytosis. We are working with the laboratory of medical genetics in La Timone. We are interested in applications for diagnosis and prediction of vasoocclusive crises.
- to understand the multi-scale response of red blood cells during splenic filtration, when the cells have to pass a stringent ‘fitness test’ in interendothelial splenic slits. We are interested in the whole process from the activation of mechano-sensitive membrane ion channels to changes in cell volume and deformability and to their ability to be transported in the microcirculation
- to understand the physics of the mucociliary We study the spatial and directional organization of the ciliary activity that generates mucus transport and the role of mucus rheology on the transdifferentiation of epithelial cells.
Centre de physique théorique (CPT)
Campus / location : Luminy
Website : http://www.cpt.univ-mrs.fr/spip.php?page=rubrique&id_rubrique=31
Team leader : Alain Barrat
Research domain : Complex networks
Contact : Alain Barrat - alain.barrat@cpt.univ-mrs.fr
Many systems of different nature and origin can be described as networks (set of nodes and links): from transportation networks to social networks, foodwebs, gene regulation networks, neural networks, etc. Despite their differences, many of these networks share common properties such as the presence of heterogeneities. It is therefore of widespread interest to characterize these properties and to understand their impact and role on processes occurring on these networks or described by these networks. We have worked in these directions, mainly in relations to (i) infrastructure networks, (ii) social networks (both online networks and real life contact networks) and in relation with epidemiological studies. Our recent focus is on temporal networks: how to characterize them, how to design models, how processes on networks are influenced by their temporal variation. While we started to study these questions in the framework of temporal contact networks between individuals and in link with epidemiology, such issues arise also in brain networks and in particular concerning functional connectivity dynamics.
Campus / location : Luminy
Website : https://centuri-livingsystems.org/m-merkel/
Team leader : Matthias Merkel
Research domain : Dynamical systems, Self-organized deformation of biological tissues, Mechanical properties of disordered systems
Contact : Matthias Merkel - matthias.merkel@univ-amu.fr
Self-organized deformation of biological tissues
During animal development and regeneration, tissues and organs grow and deform in a self-organized manner. We want to focus on oriented deformation processes, for which many examples exist throughout development. How can self-organized, oriented tissue deformation be robust? This is far from obvious, because many existing physical theories predict instabilities of such oriented actively deforming materials. We want to understand how the occurrence of such instabilities can be prevented (or even harnessed) during developmental processes and establish quantitative criteria for that.
Collaborations exist with the group of Thomas Lecuit on epithelial flows and invaginations during Drosophila embryogenesis and with the group of Pierre-Francois Lenne on gastruloid deformation.
Mechanical properties of disordered systems
How do large-scale properties of disordered systems arise from the complex interplay of their microscopic constituents? This question is important to understand a large class of materials both living and non-living. In a recent publication we have already shown that one can make surprisingly general analytical predictions about the elastic macroscopic properties of the broad class of athermal, under-constrained materials. This is relevant to understand systems as diverse as semiflexible polymer networks like collagen and cell-based models for biological tissue. We want to extend these ideas in several directions, e.g to over-constrained materials, to include finite temperatures, and to include reversible cross-linkers. This will allow us to more accurately predict the macroscopic properties of many disordered materials and directly link them to their microscopic structure.
Campus / location : Luminy
Website : http://www.cpt.univ-mrs.fr/spip.php?page=rubrique&id_rubrique=33
Team leader : Marco Pettini
Research domain : Nonlinear dynamics, biophysics, statistical mechanics, plasma physics
Contact : Marco Pettini - marco.pettini@univ-amu.fr
From the physics point of view, one of the striking and challenging problems arising from molecular biology stems from the observation that a large number of biochemical reactions and processes involving a huge number of actors (nucleic acids heteropolymers, microtubules homopolymers, and proteins) are coordinated simultaneously and efficiently sequenced in time thus giving rise to well organized dynamic systems. The right molecule has to be at the right place, at the right moment, in the right sequencing with respect to other intervening molecules in any biological elementary action. Hence it seems hard to explain the extraordinary accuracy of these molecular encounters only on the basis random diffusion. In fact, a longstanding proposal surmises that the encounters of distant cognate partners of biomolecular reactions could be actively driven by selective (resonant) attractive forces of electrodynamic nature. As these electromagnetic interactions can only be activated when biomolecules undergo out-of-equilibrium collective vibrations they can be switched on and off by suitable environmental conditions. These collective vibrations can entail strong resonant dipole interactions between biomolecules oscillating at the same frequency or with the same pattern of frequencies. This resonance mechanism would thus result in the selectivity of the interaction. For several years now we have been working on both the theoretical and experimental sides of the above surmised scenario. The experimental part has been carried on, and is still ongoing, with two teams of experimental physicists at the Institut d’Electronique des Systèmes, University of Montpellier, and of the Physics Department, University of Rome “La Sapienza”, respectively, with whom we have for the first time found an out-of-equilibrium pseudo-Bose condensation of the vibrational modes of a protein, pre-requisite for the activation of long-range electrodynamic interactions. The experimental part on the core problem of detecting the activation of these electrodynamic interactions between biomolecules has been carried on, and is still ongoing, in collaboration with two teams of biologists of the CIML (directed by P. Ferrier and D. Marguet, respectively); at present preliminary and very promising results have been found. We expect to develop this research project – which has been hitherto in the “proof of concept” phase - within CenTuri by moving in the direction of understanding whether and to what extent these phenomena actually play some relevant role in living cells. In a strictly related direction, We may propose to work also on the potential benefits of electromagnetic fields (EMFs) in the fight against cancer. Indeed, recently, and in parallel to conventional anti-proliferative treatments, there has been a steadily growing number of interesting observations and results regarding the inhibition of cancer cell growth using external EMFs, though in the absence of a convincing rationale; if a strong evidence will be found of the role of electrodynamic interactions within cells then this gap could be most likely filled, with an obvious positive impact beyond the domain of pure science.
Campus / location : Luminy
Website : https://centuri-livingsystems.org/h-rouault/
Team leader : Hervé Rouault
Contact : Hervé Roualt - herve.rouault@cpt.univ-mrs.fr
How do animals transform their visual stimuli into internal representations of objects or environments that they can use to behave in a meaningful way? Answering this question would provide a basic understanding of many cognitive abilities. Navigation relies on an internal neural representation of the external world, built using multi-sensory information. Although we live in a three-dimensional world, the modeling and analysis of navigation tasks often assume two-dimensional exploration only, limiting our understanding of neural activities. Recently, neural representations of 3D orientations have been characterized, and mechanisms of internal representations have been determined in great details using model organisms.
We propose to leverage modeling approaches and powerful theoretical tools in order to understand and conceptualize the representations animals use for their 3D spatial environment. We will attempt to establish models for the sensory network that computes the 3D orientation of scenes or objects, making use of recent advances in artificial neural networks. We will then construct neural network models able to sustain these representations independent of sensory activity. The different neural network architectures obtained in silico will be studied and compared to actual animal implementations. We will generalize our approach to the combination of orientation and spatial localization in a scene. This work will guide the interpretation of the neural activities, including the receptive fields of the neurons present in the visual system and the persistent activities observed in deeper brain areas. By understanding the space of neural activity, we will provide insight into important properties of the neural code, such as its dimensionality and how it shapes the organization of brain regions implicated in spatial representations. This more integrated view on the function of multiple interconnected brain areas will shed light on another open problem in neuroscience — how visual inputs drive the higher cognitive
Campus / location : Luminy
Website : https://centuri-livingsystems.org/jf-rupprecht/
Team leader : Jean-François Rupprecht
Research domain : biophysics, statistical mechanics, generic theoretical frameworks
Contact : Jean-François Rupprecht - rupprecht@cpt.univ-mrs.fr
The objective of the OM team is to develop generic theoretical frameworks that have a predictive value and which can inspire new experiments in biophysics. Current subjects - associated to post-doc and PhD openings - aim at answering questions inspired by embryonic development, that we model as a fluctuating hydrodynamics problem. Current active matter theories predict that viscous material can display spontaneous flows due to the local energy consumption (e.g. ATP consumption by cells). We propose to tackle the following questions: within developing embryonic tissues, what tames the generic active matter instability into well-controlled flow patterns, eventually leading to functional organs with well-controlled structures? are these patterns robust to cell-scale fluctuations? conversely, could these fluctuations play a role during embryogenesis ?
We think of these questions as exciting opportunities to explore the relation between force generation processes at the microscopic scale (e.g. molecular motor contraction at the cellular scale) and emerging mechanical properties at the macroscopic scale (e.g. embryonic tissue), with the objective of gaining a new perspective on what drives pattern formation either in vivo (embryogenesis) or in artificial systems (such as cell monolayers, cancer spheroids or organoids).
Dynamics and nanoenvironment of biological membranes (DyNaMo)
Campus / location : Luminy
Website : https://sites.google.com/view/fm4b-lab
Team leader : Felix Rico
Research domain : Biophysics, biochemistry and cell biology, nanotechnology
Contact : Felix Rico felix.rico@inserm.fr
DyNaMo is an interdisciplinary research unit with an important international openness, bringing together cell biologists, biochemists and biophysicists.
Cell membranes delineate compartments and therefore play a role of interface that is key for transmission of physical and biochemical information to and from the cell nanoenvironment. This role is dynamically regulated through reorganization and remodeling of the lipid and protein membrane composition and its interaction with cellular and extracellular components. We aim at understanding the fundamental interplay between physical, biochemical and molecular mechanisms of cell function, focusing on the components of the membrane and its nanoenvironment.
We integrate classical biochemical and cell biology approaches with the development of new force microscopy based nanotools to map the dynamics and mechanics of cell interactions. This interdisciplinary approach is developed at different scales, from the nanoscale of proteins and membranes to the microscale of cells and tissues, and at different timescales, from microseconds to minutes.
Institut Fresnel
Campus / location : Campus de St Jérôme
Website : http://www.fresnel.fr/spip/spip.php?article1102
Team leader : Hervé Rigneault
Research domain : Biophotonics, optical engineering
Contact : Hervé Rigneault - herve.rigneault@fresnel.fr
Mosaic is an interdisciplinary research group aiming at unravelling life science problems using advanced photonic tools. Mosaic principal investigators are physicists and biologists working together at the cross roads between advanced optical imaging, nanophotonics and tissue morphogenesis. In parallel, the Mosaic group is involved in collaborative projects related to the fields of developmental biology, immunology, neurosciences and biomedical research. Mosaic PI’s expertise encompass physics, optical and electrical engineering and biology. They will be able to participate in the CenTuri training program mostly on the physics part but also in Biology. Living systems, their investigation and there understanding are central in the Mosaic group research. Using dedicated custom build microscopes and endoscopes systems the group is investigating biological systems from molecules to cells and tissues. Key technologies available at Mosaic are label free nonlinear microscopy, fluorescence diffusion analysis, thermal microscopy and phase microscopy. A strong expertise is also present in the field of polarization resolved microscopy to image molecular order in living systems. Mosaic has strong commitment with industries working in the field of optical instrumentation. The group has a long standing expertise in patenting and develops the original concept of ‘co-conception’, which is the design of an instrumentation, its associated algorithms to achieve a task that is only driven by the end user. The result are unique machines optimized for a specific task from its early conception.
Institut de Recherche sur les Phénomènes Hors Equilibre (IRPHE)
Campus / location : Technopôle de Château-Gombert
Website : https://www.irphe.fr/~eloy/
Team leader : Christophe Eloy
Research domain : Biofluiddynamics, animal locomotion, wind-plant interactions, capsule and vesicle dynamics
Contact : Christophe Eloy - christophe.eloy@irphe.univ-mrs.fr
Our research addresses various fundamental problems of fluid and solid mechanics, including fluid-structure interactions, hydrodynamic instabilities, animal locomotion, aeroelasticity, rotating flows, and plant biomechanics. It generally involves a combination of analytical modeling, experiments, and numerical work.
Christophe Eloy is also Responsable des programmes (Head of programs) at the Ecole Centrale Marseille (about 300 students per year). This engineering school delivers a Master of Engineering degree. Some students may be interested in attending courses in biophysics or bioengineering, or pursue a PhD in these fields.
Campus / location : Technopôle de Château-Gombert
Website : https://www.irphe.fr/?q=fr/-Matiere-molle-et-biophysique-
Team leader : Marc Leonetti
Research domain : Electrophysiology, theroy, numerics and experiments
Contact : Marc Leonetti - leonetti@irphe.univ-mrs.fr
Biological electric activity can exhibit complexity and pattern formation called also morphogenesis in other contexts even at the cellular scale: for original patterns as they are quasi-stationary contrary to other nonlinear moving structures such as action potential or 2D cardiac spirals. Understanding patterns need a nontrivial formalism adapted to all biological configurations, namely those involving strong gradients of ionic concentrations not taken into account in the classic cable model, migration of membrane proteins, bulk chemical reactions and whatever the geometry. Indeed, some configurations need to understand the cooperation between neighbouring cells to explain negative and positive group effect. All this theoretical and numerical formalism could be applied to complex geometries such as “small” networks. Another development could the coupling between electric activity and shape deformations with mechanosensitive signalling. Marc Leonetti is also Responsable des programmes (Head of programs) at the Ecole Centrale Marseille (about 300 students per year). This engineering school delivers a Master of Engineering degree. Some students may be interested in attending courses in biophysics or bioengineering, or pursue a PhD in these fields. Mechanics of membranes and dynamics of “soft microparticles”: Improving drug delivery, cosmetic and nutrition motivates one part of this work to model the behavior of soft microparticles such as liposomes, microcapsules, polymersomes and protein aggregates in flow. Behavior means questioning the shape, its spatiotemporal dynamics, its membrane phase transitions under constraints and finally its breakup. Our team performs experimentally these studies with microfluidic tools and theoretically with the development of an unique numerical model (coll. M2P2) able to describe a large range of membrane materials from a fluid lipid bilayer to a thin viscoelastic network, two key ingredients of red blood cell membrane in fact. Note that we attach a great importance to the quantitative comparison between results from experiments and numerics/theory. Experiments are performed on single “soft microparticle” and on suspensions to study the phenomenon of margination in vessels for example. The second motivation concerns the behavior of biological cells under constraints when they are isolated or in dense suspensions such as in blood or lymph. One question is how these typical suspensions flow ? Is it optimal at a biological point of view ? Is it possible to detect (and separate) sick cells from their behavior in flow ? to predict “dead” domains in vasculatory branching network ? One specificity of our approach is to capture spatial multiscale problematics with aspect ratio up to 100-500. It means that the cellular shape under local forces induced by molecular motors and actin polymerization for example could be modelled.
Institut Universitaire des Systèmes Thermiques Industriels (IUSTI)
Campus / location : Technopôle de Château-Gombert
Website : https://iusti.cnrs.fr/en/
Team leader : Olivier Pouliquen
Research domain : Physics, mechanics, biomechanics
Contact : Yoel Forterre - yoel.forterre@univ-amu.fr; Olivier Pouliquen - olivier.pouliquen@univ-amu.fr
Our research focuses on the behavior and dynamics of complex fluids and soft matter. Examples are flows of granular media, of suspensions, behavior of gels and poroelastic media. To understand the underlying physical mechanisms and propose constitutive models, we combine experiments on simplified systems and theoretical modeling.
Recently, we extend this research to biological systems by addressing questions of mechanosensing, signaling and motion in plants, both at the organ and cellular level.
Examples of problems are :
-How plants feel and react to change in gravity conditions (gravitropism)
-How plants feel and react to mechanical stimuli, for example bending (thigmo response)
-How fast motions can be generated in plants without muscles (venus flytrap for exemple).
Laboratoire Adhesion & Inflammation (LAI)
Campus / location : Luminy
Website : https://labadhesioninflammation.org/
Team leader : Olivier Théodoly
Research domain : Biophysics, immunology, cell migration, molecular interactions
Contact : Olivier Theodoly - olivier.theodoly@inserm.fr
LAI’s main research domain concerns Biophysics of the immune response. The LAI is a multidisciplinary research unit composed of investigators with backgrounds in physics, biology or medicine. We intend to adapt physical concepts and methodologies to achieve a quantitative understanding of cell function, notably cell adhesion and migration in the immune system, with the goal of applying the results of our fundamental research to relevant clinical problems. The main thematics at LAI concern T lymphocyte immune response at different length scales, from the molecule to the tissue: molecular level (molecular recognition of antigens by immune cells), cellular level (Mechanisms of adhesion, activation, migration and guiding of single cells) and tissue level (reorganization of lymphatic vessels in inflammation zones).
Laboratoire de Mécanique et d'Acoustique (LMA)
Campus / location : Luminy
Website : http://www.lma.cnrs-mrs.fr/spip/spip.php?auteur14
Research domain : Ultrasonic backscattering, quantitative ultrasound, sheared concentrated suspensions, ultrasound neuromodulation
Contact : Emilie Franceschini - franceschini@lma.cnrs-mrs.fr
Our research aims to probe complex biological media (sheared concentrated suspensions of red blood cells and cancerous tumors), and to activate neurons by ultrasound. Our main projects are:
- To characterize red blood cell aggregation processes and the structure of aggregates in blood by using a cellular ultrasound imaging modality. This imaging modality is a novel tool in fluid mechanics for investigating the rheological properties of concentrated (opaque) suspensions. The clinical application is the monitoring of inflammatory response accompanied by abnormal levels of aggregation for patients in septic shock or for post-operative sepsis follow-up.
- To understand the ultrasonic scattering from cells undergoing cell death toward the monitoring of cancer therapy. The aim is to characterize the acoustic/mechanical properties from single cells undergoing cell death process in order to investigate the cellular scattering sources from an ensemble of heterogeneous cells (micro/macro link).
- To understand the physical and molecular mechanisms of ultrasound-induced neurostimulation. One potential advantage of focused ultrasound compared to electrical stimulation is the ability to selectively target restricted subpopulations of neurons. This feature makes ultrasound neurostimulation a promising alternative tool for the design of future neural prostheses: for example, to restore hearing in deaf people, because contemporary cochlear implants – which work via electrical stimulation - mainly suffer from a lack of spatial selectivity. This project is conducted in collaboration with the group of A. MOQRICH, expert in molecular and cellular biology at IBDM (CENTURI).
Laboratoire de Mécanique, Modélisation & Procédés Propres (M2P2)
Campus / location : Technopôle de Château-Gombert
Team leader : Marc Jaeger
Contact : Julien Favier - julien.favier@univ-amu.fr
The team is developing an expertise in numerical simulation and predictive flow analysis in application domains centered on aeronautics, fusion, lung flows and hydrodynamic transfers. Innovative and optimized numerical methods are developed to respond to fundamental scientific challenges, industrial applications and current societal issues.
The team currently includes 10 researchers and teacher-researchers, and is structured around 4 research axes:
- Aerodynamic flows
- Biological flows
- Flows for magnetic
- Hydrodynamic fusion and parietal transfers
Campus / location : Technopôle de Château-Gombert
Website : http://www.m2p2.fr/
Team leader : Marc Jaeger
Research domain : Fluid-structure interactions at microscale (soft biomimetic particles, cilia, …)
Contact : Marc Jaeger - marc.jaeger@centrale-marseille.fr
At the microscale, mass transfer is dominated by surface and interfacial phenomena. For soft interfaces, as is found in living systems, the interplay between the microfluidics and the mechanics of interface (fluid-structure interaction) produces highly non-linear hydrodynamic interactions leading finally to the emergence of some complex organisation. The rheology of complex fluids like blood and lymph is the result of such underlying organisation. This fundamentally physical base is exploited by nature to optimize the transport inside living systems. The transport can be that of a physiological fluid by a ciliated epithelial tissue like in mucociliary clearance or that of a whole microorganism on which cilia or flagella are attached. It can also take the form of encapsulated trafficking like transport of oxygen by red blood cells in the blood circulation. In the first case the structure is a 1D soft slender body whereas in the second it’s a 2D soft membrane that can be treated as continuous material lines or surfaces imbedded in a 3D bulk fluid. Understanding the dynamics of such fluid-structure systems needs to represent correctly the mechanical answer of the soft micro-structures to the hydrodynamic stress and maybe some other physicochemical stresses. In our group, we develop original numerical models and methods to handle this kind of problems. We collaborated with other teams inside or outside of our lab, to couple with experimental studies on the same subjects for example. Now applying our expertise in numerical and mathematical modelling of soft matter and complex fluids to real biological problems needs to collaborate with biologists At the microscale, mass transfer is dominated by surface and interfacial phenomena. For soft interfaces, as is found in living systems, the interplay between the microfluidics and the mechanics of interface (fluid-structure interaction) produces highly non-linear hydrodynamic interactions leading finally to the emergence of some complex organisation. The rheology of complex fluids like blood and lymph is the result of such underlying organisation. This fundamentally physical base is exploited by nature to optimize the transport inside living systems. The transport can be that of a physiological fluid by a ciliated epithelial tissue like in mucociliary clearance or that of a whole microorganism on which cilia or flagella are attached. It can also take the form of encapsulated trafficking like transport of oxygen by red blood cells in the blood circulation. In the first case the structure is a 1D soft slender body whereas in the second it’s a 2D soft membrane that can be treated as continuous material lines or surfaces imbedded in a 3D bulk fluid. Understanding the dynamics of such fluid-structure systems needs to represent correctly the mechanical answer of the soft micro-structures to the hydrodynamic stress and maybe some other physicochemical stresses. In our group, we develop original numerical models and methods to handle this kind of problems. We collaborated with other teams inside or outside of our lab, to couple with experimental studies on the same subjects for example. Now applying our expertise in numerical and mathematical modelling of soft matter and complex fluids to real biological problems needs to collaborate with biologists
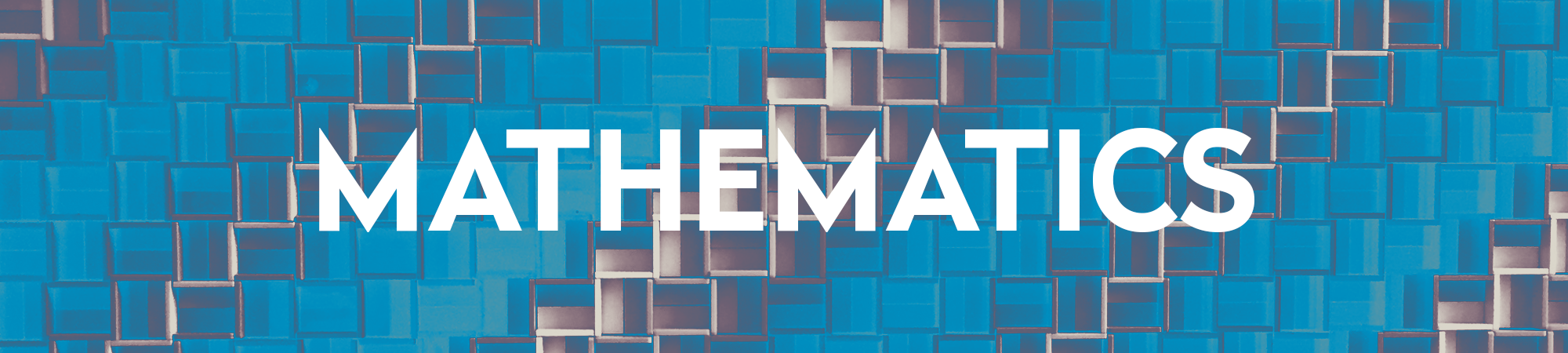
Institut de Mathématiques de Marseille (I2M)
Campus / location : Technopôle de Château-Gombert
Website : http://www.i2m.univ-amu.fr/Equipe-Analyse-Appliquee-AA
Team leader : Florence Hubert
Research domain : Applied mathematics
Contact : Florence Hubert - florence.hubert@univ-amu.fr
Our main research domain concerns biological modeling based on partial differential equations (PDE). We have been working for a longtime with biologists, pharmacologists, physicians. One of our collaboration is already involved in the CENTURI network and we wish to intensify our participation through new collaborations. Here are some example of ongoing projects in our group :
- Interactions with Institut National de Recherche en Agronomie in Avignon
Team : M. Cristofol, F. Hamel , collaboration with L. Roques. Keywords : Inverse problems in parabolic PDEs modeling diffusion in biological systems, evolution of populations, diffusion of genes…. Biological invasions, adaptative dynamics, selection-mutation.
- Interactions with the Center for Research in Oncolobiology and oncopharmacology
Team : A. Benabdallah, G. Chapuisat, C. Gomez, F. Hubert, M. Tournus, collaboration with N. André, D. Barbolosi, M. Carré, J. Ciccolini, S. Honoré Keywords : Models of metastatic spreading, impact of combined therapy (Developpment of news indicators of the metastatic state of patients, a step towards individual optimized therapies) Models of tumor growth under metronomic therapy. (A step at the cell level for a better understanding of the metronomic paradigm) Models at the cell level the resistance to chemotherapy. (A step for a better understanding of the chemotherapy resistance with the aim of designing new protocoles) Models for the dynamical instability of microtubules with the possibility to involve microtubule targetting agents. (A way to get a better insight of the low dose action of the drug at the mesosscopic level )
- Interaction with physicians in APMH timone, Marseille
Team : F. Hubert Keywords : Metastatic melanoma under anti-BRAFi (coll with Pr Grob group) Modeling the kinetics of thrombin generation (Coll with Pr PE Morange)
- Interaction with Cancer Research Center of Marseille
Team : F. Hubert, M. Tournus coll. with with C. Acquaviva, and E. Mamesier Keywords : Metastases spreading and circulating tumors cells
- Interaction Adhesion & Inflammation Lab
Team : F. Hubert, J. Olivier collaboration with O. Theodoly Keywords : models cell migration in confined domains
Campus / location : Technopôle de Château-Gombert
Website : http://www.i2m.univ-amu.fr/
Team leader : Pierre Pudlo
Research domain : Statistics (theoretical, computational and applied)
Contact : Elisabeth Remy - elisabeth.remy@univ-amu.fr
Despite its small size, the statistical team of the mathematical unit I2M is interested in a wide range of subjects.
A first half of our group is focused on theoretical properties of data processing algorithms whose tuning parameters are automatically adapted on the data itself. Some of the algorithms we study (to show that they are optimal or almost optimal) are Lasso or Dantzig-selector regression, nonparametric regression, and so on. Other algorithms that we study are indeed statistical tests on high dimensional data.
The second half of our group is more applied and studies various topics such as:
- Multiple tests
- Multivariate extremal analysis
- Statistical modeling of the risk
- Times series and long memory process
- Bayesian data analysis (multivariate regression, statistical models with a high number of unobserved variables) with MCMC (Markov Chain Monte Carlo) algorithms or ABC (Approximate Bayesian Computation)
- Statistical analysis of data on graphs with Markov random fields
- Unsupervised learning and data clustering.
Recently recruited researchers, that have experimented inter-disciplinary work, may represent an opportunity to focus part of our research on the design of statistical model and efficient statistical algorithms for system biology.
Campus / location : Luminy
Website : http://www.i2m.univ-amu.fr/
Team leader : Elisabeth Remy
Research domain : Biological networks - exploration, modelling and analysis
Contact : Elisabeth Remy - elisabeth.remy@univ-amu.fr
MaBios (Mathématiques et Algorithmique pour la Biologie des Systèmes) is an interdisciplinary team of the Institut de Mathématiques de Marseille (I2M).
Our group is composed of CNRS researchers and AMU assistant professors trained in different scientific fields: mathematicians, computer scientists and bioinformaticians.
Our main goal is to develop innovative network-based approaches to investigate biological interaction networks, following two main axes:
- Modelling and analysis of dynamical regulatory networks:
- Modelling specific biological processes and study impact of given perturbations, such as disease-induced perturbation;
- Studying dynamical properties of discrete logical models, and structural properties of the regulatory network;
- Exploiting large-scale interaction networks to study the genotype-phenotype complexity
- Studying gene and protein cellular functioning thanks to community-detection algorithms;
- Predicting disease-associated genes thanks to local network explorations.
To undertake these tasks, we rely on concepts coming from different mathematical fields such as graph theory, discrete dynamical systems, logic, stochastic processes, combinatorial algorithmics. We further combine our different backgrounds while remaining closely focused on biological applications
Campus / location : Technopôle de Château-Gombert
Website : http://www.i2m.univ-amu.fr/
Team leader : Frédéric Richard
Research domain : Mathematics applied to signal, image processing
Contact : Elisabeth Remy - elisabeth.remy@univ-amu.fr
The Signal and Image team at I2M specializes in developping mathematical tools and models for signal and image processing problems. The main mathematical research themes are
- Statistical models, non-stationary random processes and applications,
- Variationnal methods, optimisation and applications,
- Multiresolution, time-frequency analysis and approximation.
There is furthermore a close collaboration with the Statistical Learning team QARMA of the LIF.
Campus / location : Campus Luminy
Website : www.i2m.univ-amu.fr/user/philippe.roudot/
Team leader : Philippe Roudot
Contact : Philippe Roudot - philippe.roudot@univ-amu.fr
Our research focuses on the automatic identification of collective molecular processes in biomolecular clouds, establishing the acquisition parameters required to detect them and their exploration in bioimaging datasets of ever-growing size. This is a challenging problem because molecular clouds are dense and composed of a mixture of multiple concurrent processes that must be detected and tracked independently (e.g. membrane motions, signal events, chromosome capture…). The large number of possible associations makes their identification a NP-hard problem. At the same time, the local dynamics are represented by the combination of a stochastic component (e.g. tethered diffusion), a functional component (e.g. nucleation, turnover) and a structural component (e.g. membrane or embryo deformation,). To tackle these challenges, we are developing new approaches dor i) multiscale dynamic modeling for biomolecular clouds using both conventional stochastic and deep neural network modeling, ii) efficient optimization approaches to identify them into collective processes and iii) new software for efficient rendering and data access.
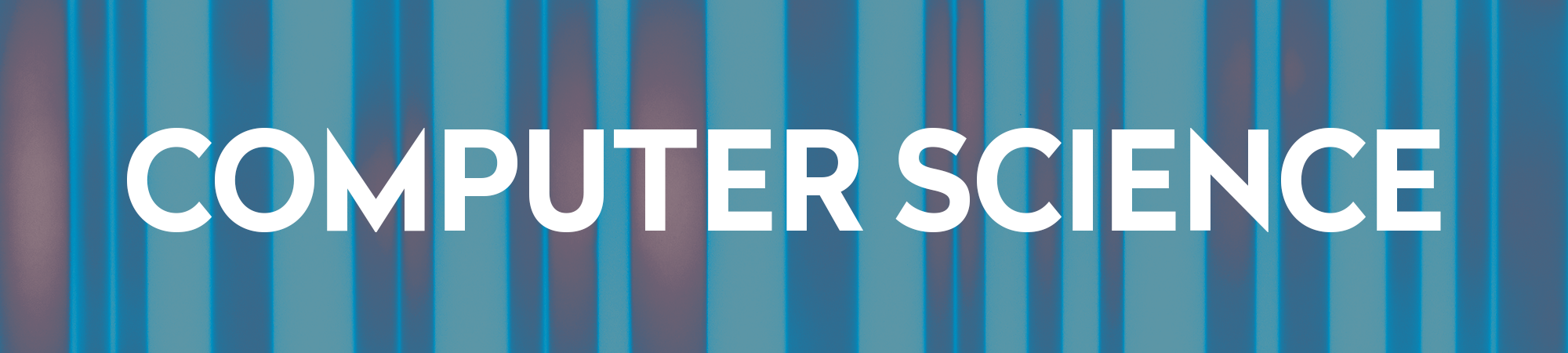
Laboratoire d’Informatique et Systèmes (LIS)
Campus / location : Luminy
Website : https://www.lis-lab.fr/lirica/
Team leader : Vincent Risch
Research domain : Logic, Reasoning, Complexity, Knowledge Representation, Artificial Intelligence, Semantics, Proof Theory, Formula Satisfiability
Contact : Belaid Benhamou- belaid.benhamou@univ-amu.fr
The LIRICA team (Logic, Interaction, Reasoning and Inference, Complexity, Algebra) is part of the two main lines of the LIS Computing pole: Logic and Formal Methods on the one hand, and Artificial Intelligence on the other. Their research focuses on the formalization of different types of Reasoning and Interactions, the theory of Complexity, Algebraic systems and tools, these three axes being enriched by multiple interactions.
Campus / location : Saint Jérôme Campus
Website : https://www.lis-lab.fr/diams/
Team leader : Omar Boucelma & Noel Novelli
Research domain :Data analysis and data mining, Integrated management of heterogeneous and multimodal data, Composition of analytical processing chains and services, Optimization and customization
Contact : Omar Boucelma - omar.boucelma@lis-lab.fr; Noel Novelli - noel.novelli@lis-lab.fr
The data we are interested in is typically heterogeneous, massive, rapidly evolving, multi-modal, and often dirty with errors such as missing values, duplicates, uncertainties, and outliers. It is extracted from the Web, social and wireless networks, connected objects, sensors and scientific applications.
Our main application domains are: Healthcare, Sustainable Development and Environmental Sciences, Defense, Maritime Transport, Smart Cities and IoT, leveraging our fruitful collaborations with our partnering industries and scientific institutions.
Campus / location :
Website : http://www.lsis.org/mofed
Team leader : Isabel Demongodin
Research domain : Algorithms, distributed algorithms, calculation models, mobile agents, shared memory, dynamic networks
Contact : Isabel Demongodin - isabel.demongodin@lsis.org
The MoFED project focuses on the development of methodologies for modelling, analysis and control of event-driven systems. Discrete Event Specifications (DEVS), Petri nets, PLCs and networked system models are the preferred paradigms of study.
We find in different fields, the same classes of models with more or less different analysis techniques, whether in the field of computer science, more precisely in the specification and verification of reactive or real-time systems, or in the field of automation and productivity for the study of control systems or performance evaluation. Any design or operating stage of a dynamic system (computer system or automated system) must include the various stages of specification, validation and implementation, and must also manage the constraints related to distribution (resource sharing) and synchronisation (communication). Although the choice of model(s) depends on the system being designed and its properties to be analyzed, it is often necessary to combine models and analysis tools to determine both behavioral and structural properties.
In this multidisciplinary context, the problem-solving methods developed in this project are of a different nature, including those based on simulation and analytical approaches (optimization, formal analysis, numerical analysis).
More specifically, the themes developed in this research project concern :
- theoretical contributions in discrete event formalisms (extensions, gateways, etc.),
- the acquisition and representation of knowledge and reasoning for the construction of models,
- conceptual modelling and simulation architectures,
- the integration of formal verification methods with simulation methods,
- analysis and optimization of event-driven models of networked systems.
Website : https://www.lis-lab.fr/im/
Team leader : Pierre Drap
Research domain : Image analysis, medical imaging, vision, telemonitoring, photogrammetry, classification, learning, pattern recognition, decision support, optimization, simulation
Contact : Pierre Drap - pierre.drap@lis-lab.fr
The Images & Models team of the LSIS is an image analysis team whose activities aim at extracting knowledge and decision support from image data. These activities are therefore oriented towards :
- diagnostic assistance
- pre-operative planning
- behavioural analysis
- morphometrics
- information systems
The methodological fields addressed to achieve these objectives are :
- computer vision and photogrammetry techniques.
- pattern recognition, based on the extraction of local and global descriptors and the use of partitioning (clustering) and learning techniques.
- analysis of 3D geometric shapes, in particular in terms of description and characterization, representation, metrics and similarities, parameterization, and surface registration.
Finally, the techniques and tools used can be categorized in :
- low-level techniques such as filtering, segmentation, resetting.
- processing of data structured in graphs: graph matching, processing of data defined on meshes.
- classification, in the broadest sense.
Campus / location : Luminy
Website : http://www.lis-lab.fr/dalgo/
Team leader : Jérémie Chalopin
Research domain : Algorithms, distributed algorithms, calculation models, mobile agents, shared memory, dynamic networks
Contact : Emmanuel Godard - emmanuel.godard@lis-lab.fr
The DALGO team is concerned with the study of distributed and decentralized systems with a focus on the algorithmic aspects. In particular we are interested in the computational power of various models for distributed computing and the communication complexity of distributed solutions to fundamental problems in these models. Members of our team work on the following themes of research:
- Design and Analysis of Distributed Algorithms
- Distributed Computing with Mobile Agents or Mobile Robots
- Dynamic Network Models
- Embedded Systems and Synchronous Programming Languages
Campus / location : Chateau-Gombert
Website : https://qarma.lis-lab.fr/
Team leader : Hachem Kadri
Research domain : Automatic Learning, dictionary learning, deep learning, representation learning, spectral learning
Contact : Cécile Capponi - cecile.capponi@lis-lab.fr
Qarma has developed a general expertise in machine learning and modern artificial intelligence, from theory and algorithms to applications.The team is composed of about 15-20 members with complementary skills covering all fields of machine learning, as illustrated by Qarma’s training activities.
Research works at Qarma are currently focussed on three major axes :
- learning theory
- deep learning
- connections between machine learning and signal processing
and two transverse axes closely related to the above major axes :
- multi-view learning
- machine learning for neurosciences
Qarma’s research has also been related to spectral learning, grammatical inference, multi-task learning, active learning, budget learning, scalability, applications to gesture, audio, video, image, language.
Campus / location : Luminy
Website : http://www.lis-lab.fr/move/
Team leader : Pierre-Alain Reynier
Research domain : Distributed system, verification, logic, automata and combinatorics
Contact : Jean-Marc Talbot - jean-marc.talbot@lis-lab.fr
The concept of distributed system is fundamental both for practical applications and theoretical foundations of computer science. The conception of applications safe and sound in this context is especially hard. The research of MoVe team is focused on this problem and our objective is to contribute on fundamental concepts in this area, and also to develop tools to validate our results. Our research topics are gathered according to two main axes: Verification and Logic. The team is involved in teaching in master of Computer Science.
Verification:
How to design formal models for systems (programs, distributed systems,..) and design efficient algorithms to verify properties of these systems.
- Extension of formalisms for the modelization and analysis of perturbations
- Synthesis of systems from MSC specifications
- Algorithmic and software development
- Structured models
Logic, automata and combinatorics:
Study the logic problems issued from verification, with a focus on problems related to expressiveness and semantics
- Descriptive complexity
- Automata and fixpoints
Campus / location : Luminy
Website : http://www.lif.univ-mrs.fr/recherche/equipes/8/presentation
Team leader : Sylvain Sené
Research domain : Discrete mathematics, natural computating, complexity, computability, biological modelling
Contact : Sylvain Sené - sylvain.sene@lif.univ-mrs.fr
Natural computation is a field of computer science. It is based on the links between informatics and other disciplines, mainly physics and biology. On the one hand, it aims at abstracting natural phenomena in order to develop new computational paradigms, or deepening the study of well-known nature-inspired models of computation. On the other hand, it aims at using these models of computation in order to achieve a better understanding of the phenomena at hand, with the hope to unravel some of the underlying fundamental laws. The CANA research group (CANA is for "CAlcul NAturel", a French acronym for natural computation), in particular, seeks to capture at the formal level some of the fundamental paradigms of theoretical biology and physics, via the models and approaches of theoretical computer science and discrete mathematics. These approaches and their underlying methods, that we develop also per se, rest on the study of interacting entities. Most often in the models that we study, each of the constitutive entities is elementary and can only take a finite number of states. Moreover, they interact only locally with respect to the network on which they lie in discrete time steps. In spite of their apparent simplicity, these discrete models are able to capture the essence of the dynamics of numerous natural systems, upon which they shed a new light. For instance, thanks to their high level of abstraction, these models provide a qualitative representation of biological regulation networks, of particle propagation in quantum physics, or even of the emergence of waves in sandpiles. Beyond their ability to grasp natural phenomena, the models are also being studied for their own sake, in terms of their intrinsic dynamical, computability and complexity properties. To define nature-inspired discrete models; to demonstrate their relevance; to tame the complex behaviours that they generate by means of rigorous mathematical results; to use this to better understand real systems: these are the main concerns of CANA research team. Depending on the situation, the important features of the model can be of static (syntactic) or dynamical (semantic) nature. Moreover, the network of entities may be regular or not, the interaction rules may be deterministic, probabilistic, synchronous or asynchronous... We study these models and their variations both for their representational and computational abilities. The methods used come from discrete dynamical system theory, combinatorics, complexity and computability theories, and non-monotonic logic, linear algebra, and quantum information.
Campus / location : Luminy
Website : https://centuri-livingsystems.org/p-villoutreix/
Team leader : P. Villoutreix
Research domain : Embryogenesis, microscopy imaging datasets, deep learning, representation learning, spectral learning
Contact : Paul Villoutreix - paul.villoutreix@univ-amu.fr
Embryogenesis is the process by which a single fertilized egg gives rise to a multicellular organism. This remarkable transformation involves the dynamic interplay of processes at multiple scales of biological organization, from molecular components and molecular machines, to single cells, tissues, and organs. The understanding of how an embryo forms is at the foundation of our comprehension of many fundamental processes, such as evolutionary dynamics, reproduction, tumorigenesis, or the formation of the immune system. Studies of dynamics in developing embryos are also driving the design of man-made tissues and organs and inspire the synthesis of active materials. Many open questions remain in our understanding of how the shape of multi-cellular systems emerges from and regulates molecular dynamics of gene expression within cells. Answering them requires harnessing massive microscopy imaging datasets and developing new mathematical methods designed for specific applications of developmental biology.
Paul Villoutreix's team is part of the QARMA team.
Campus / location : Luminy
Website : https://centuri-livingsystems.org/l-guignard/
Team leader : Léo Guignard
Research domain : Computer science, biology
Contact : Léo Guignard - l.guignard@uiv-amu.fr
Embryos develop following a predefined set of rules, in a reproducible manner to allow the organisation of a functioning organism.
We are interested in applying Computer Science to quantitatively characterise and understand this developmental reproducibility across spatial and temporal scales.
We are pursuing two main research axes:
-
Building average representatives of a population of developing embryos
-
Characterising developmental variability across spatio-temporal scales
Marseille Medical Genetics (MMG)
Campus / location : La Timone Faculty of Medecine
Website : https://www.marseille-medical-genetics.org/n-levy/a-baudot/
Team leader :Anaïs Baudot
Research domain : Network and Systems Biology, Computational Biology, Bioinformatics, Applications to human genetic diseases
Contact : Anaïs Baudot -anais.baudot@univ-amu.fr
The group Networks and Systems Biology for Diseases focuses on untangling the genotype-phenotype relationships of human disorders. Disease complexity is the consequence of the intricate cellular functioning of genes and proteins. Indeed, biological molecules do not act alone, but rather interact with each other to perform their functions in protein complexes, signalling pathways or metabolic reactions. The disease phenotypes are thus resulting not from perturbations of isolated genes or proteins, but of complex networks of molecular interactions.
In this context, thanks to network modeling and large-scale interactome and -omics mining, our group studies genes and proteins in their cellular contexts, towards a better understanding of their perturbations in diseases. We further investigate the relationships between different diseases, in particular disease comorbidities, and aim to improve diagnostic and therapeutic predictions.
Theories and Approaches of Genomic Complexity (TAGC)
Campus / location : Luminy
Website : https://tagc.univ-amu.fr/
Team leader : Pascal Rihet
Research domain : Bioinformatics, genomics
Contact : Jacques van Helden - Jacques.van-Helden@univ-amu.fr; Christine Brun - christine-g.brun@inserm.fr
Main research domains Development of bioinformatics and experimental approaches to address the complexity of biological systems, with a specific focus on genome-wide approaches to biological function and regulation. Objectives
- Go beyond holistic enumeration of elements and features extracted from massive datasets, to bridge the gap between data production and a real understanding of biological systems, i.e. decipher the causal relationships between genotype, epigenotype and phenotype at different levels of living systems.
- Develop novel approaches to address current challenges regarding the interconnection between omics data, theoretical models and biological knowledge.
Problems of interest
- Analysis of large-scale data, their representation and integration.
- Regulation of gene expression: enhancers, silencers, regulatory variants, ...
- Protein functions.
- T cell differentiation and leukemia.
- Molecular mechanisms of diseases (haematological malignancies, sepsis, malaria, cardiopathies), incl. perturbations of regulatory elements and molecular networks.
- Cardiac function and regulation.
- Host-pathogen relationships, including the role of immune cells in host protection or pathogenesis.
* : CENTURI PIs
CENTURI is laureate of the National call ”Instituts Convergences” of the French State in the context of the ”Investments for the Future” programme (2nd PIA). The project will recruit more than 100 people (researchers, engineers, PhD students, Post-docs) over 9 years with a budget of € 20 million co-funded by the French National Research Agency and the A*MIDEX Foundation.